What Is Regenerative Medicine?
Regenerative medicine replaces tissue or organs that are damaged by trauma, disease, or congenital disorders. This is different from more traditional therapies that treat the symptoms of tissue damage. There are three main concentrations within the field of regenerative medicine: tissue engineering, cellular therapy, and artificial organs. The use of tissue engineering in regenerative medicine, known as TERM, is an active area of research that involves creating functional tissue through the combination of cells, scaffolds, and growth factors to restore normal biological function.1 Clinicians treat millions of patients with tissue engineered regenerative devices. So far, the most successful tissue regeneration therapies occur in soft tissues such as skin, cartilage, and corneal tissues.
Using Tissue Engineering to Regenerate Damaged Tissue
How does tissue engineering work?
During healthy tissue development, cells build and surround themselves with an extracellular matrix. This matrix, or scaffold, contains structural proteins and acts as a reservoir for signaling molecules that cells use to communicate and organize themselves into functional complexes or tissues.
The overall goal of tissue engineering in the context of regenerative medicine is to establish a 3D cell or biomaterial complex that functions similarly to the in vivo tissue extracellular matrix. In general, tissue engineering involves the design and implantation of a scaffold that is biologically compatible with the area to be regenerated. New cells are then either attracted to or grown directly onto the scaffold.2 The FDA has approved engineered artificial cartilage and skin therapies, and researchers are developing many other therapies for different tissues and disorders (see table below).
Scaffolds in tissue engineering
Scientists seed scaffolds with their desired cell type during or following implantation. Alternatively, they may add growth factors to the scaffold and wait until the structure is populated by the surrounding tissue.
Choosing a scaffold type and source for tissue engineering is imperative for regenerating functional tissue. Pore size and overall architecture are important variables to consider when designing a scaffold. Pores play a crucial role in tissue regeneration because they allow for the exchange of nutrition and oxygen with surrounding tissue as well as expulsion of waste products and vascularization. The overall architecture of the tissue is important for exposing surfaces for cell attachment as well as mechanical cell stimuli.
Scaffolds can be natural or synthetic. Natural scaffolds are derived from donor tissues where the cells are chemically removed, leaving only the extracellular matrix. Natural scaffolds can either come from a patient or a healthy donor, and they have the advantage of retaining the unique structural and functional architecture of complex tissues. Researchers can also create natural scaffolds in vitro, such as those made from collagen and Matrigel, which are comprised of basement membrane proteins.3,4
Scientists can develop synthetic scaffolds from various polymers, including polyglycolic acid (PGA), polylactic acid (PLA), and poly(lactide-co-glycolide) acid (PLGA). Scaffolds made with these polymers are flexible and porous, making them ideal structures for cellular integration. Synthetic scaffolds are also biodegradable, with different polymers degrading at different times, allowing damaged tissue to regenerate without the use of permanent prosthetic implants. Synthetic scaffolds also have consistent structures between replicates as they are generated in a laboratory; however, they can cause inflammation in the recipient more readily than natural scaffolds.5
3D printing in tissue engineering
With recent progress in 3D printing methods, researchers create complex synthetic scaffold structures with more consistent architecture and pore sizes. Hydrogel materials, such as alginate hydrogel and gelatin, are typically used in 3D printing due to their effective crosslinking and biocompatible properties.6
Stem cells in tissue engineering and regenerative therapy
Mesenchymal, embryonic, and induced pluripotent stem cells effectively promote damaged tissue regeneration. However, in many tissues, transplanted stem cells have poor survival and differentiation capabilities. The development of stem cell technology in combination with tissue engineering techniques, such as scaffolds and the addition of growth factors, has allowed researchers to improve the viability and proliferation of stem cells in regenerative medicine.7
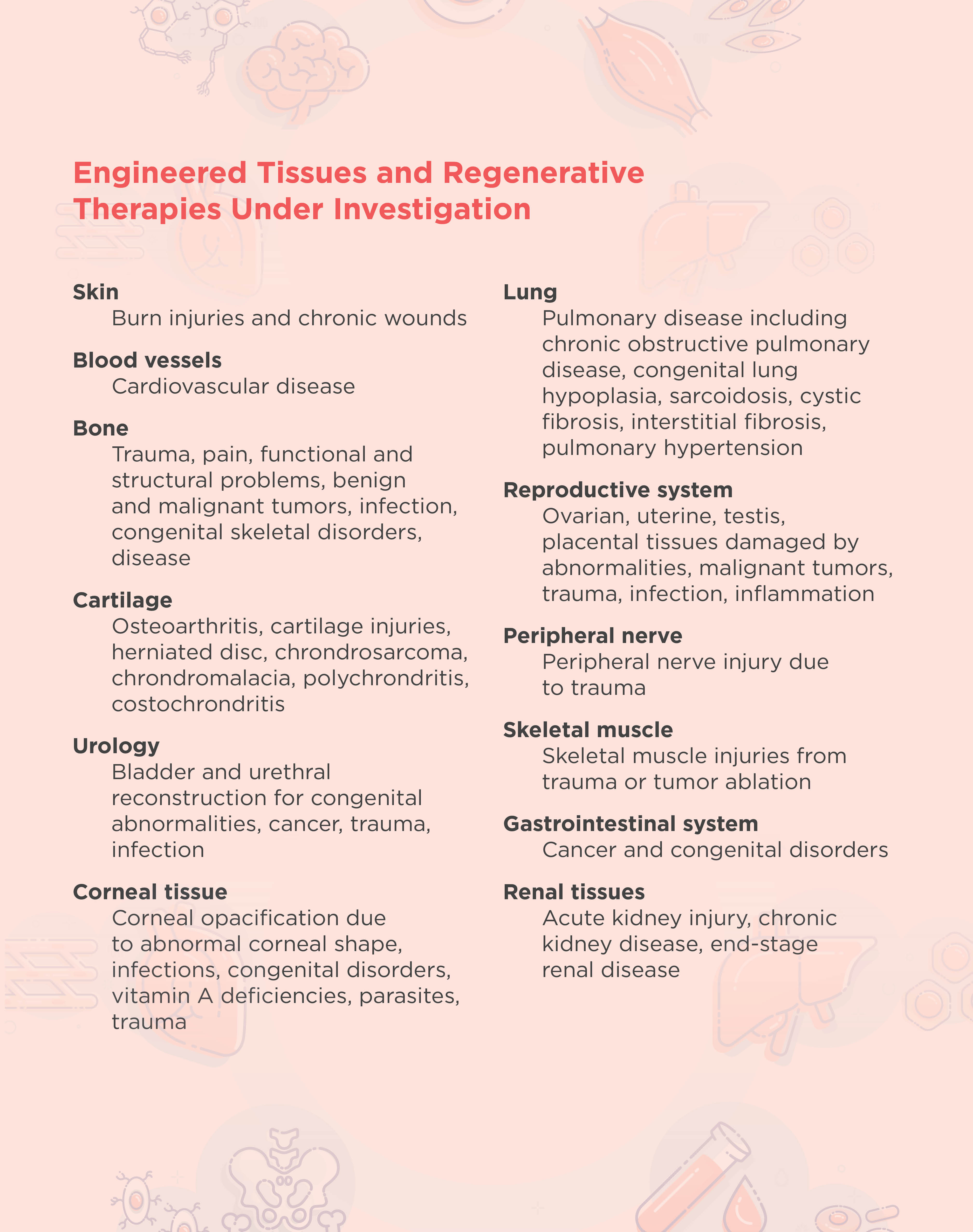
References
- A.J. Salgado et al., “Tissue engineering and regenerative medicine: Past, present, and future,” Int Rev Neurobiol, 108:1-33, 2013.
2. F. Han et al., “Tissue engineering and regenerative medicine: Achievements, future, and sustainability in Asia,” Front Bioeng Biotechnol, 8:83, 2020.
3. S. Sundaram et al., “Tissue engineering and regenerative medicine” in Rossi's Principles of Transfusion Medicine. Fifth edition. T.L. Simon, ed., New York, N.Y.: John Wiley & Sons Inc., 2016, pp. 488-504.
4. C. Motta et al., “Tissue engineering and regenerative medicine” in Rossi's Principles of Transfusion Medicine. Sixth edition. T.L. Simon, ed., New York, N.Y.: John Wiley & Sons Inc., 2022, pp. 648-660.
5. Y. Li et al., “The effect of mechanical loads on the degradation of aliphatic biodegradable polyesters,” Regen Biomater, 4:179-190, 2017.
6. Z. Yazdanpanah et al., “3D bioprinted scaffolds for bone tissue engineering: State-of-the-art and emerging technologies,” Front Bioeng Biotechnol, 10:824156, 2022.
7. S.G. Kwon et al., “Recent advances in stem cell therapeutics and tissue engineering strategies,” Biomater Res, 22:36, 2018.
