ABOVE: Pierre Agostini (left), Ferenc Krausz (center), and Anne L’Huillier (right) won the Nobel Prize for generating experimental methods for the study of attosecond physics. Ill. Niklas Elmehed © Nobel Prize Outreach
Anne L’Huillier of Lund University, Pierre Agostini of The Ohio State University, and Ferenc Krausz of the Max Planck Institute of Quantum Optics won this year’s Nobel Prize in Physics “for experimental methods that generate attosecond pulses of light for the study of electron dynamics in matter,” the Nobel Committee for Physics at the Royal Swedish Academy of Sciences announced today (October 3). Their discoveries, which capture electrons in flashes of light, have future applications in medical diagnostics, catalysis, and electronics.
Eva Olsson, chair of the 2023 Nobel Committee for Physics, began the press conference this morning with an explanation of attosecond physics. A heartbeat is one second long. Divide that by 1,000, and then by 1,000 another four times. That is the time it takes an atom to move. By dividing that time again by 1,000, physicists enter attoseconds and the world of electrons. “The ratio of one second to an attosecond is the same as the ratio of the age of the universe expressed in seconds to one second,” said Mats Larsson, a member of the 2023 Nobel Committee for Physics, during the press conference.
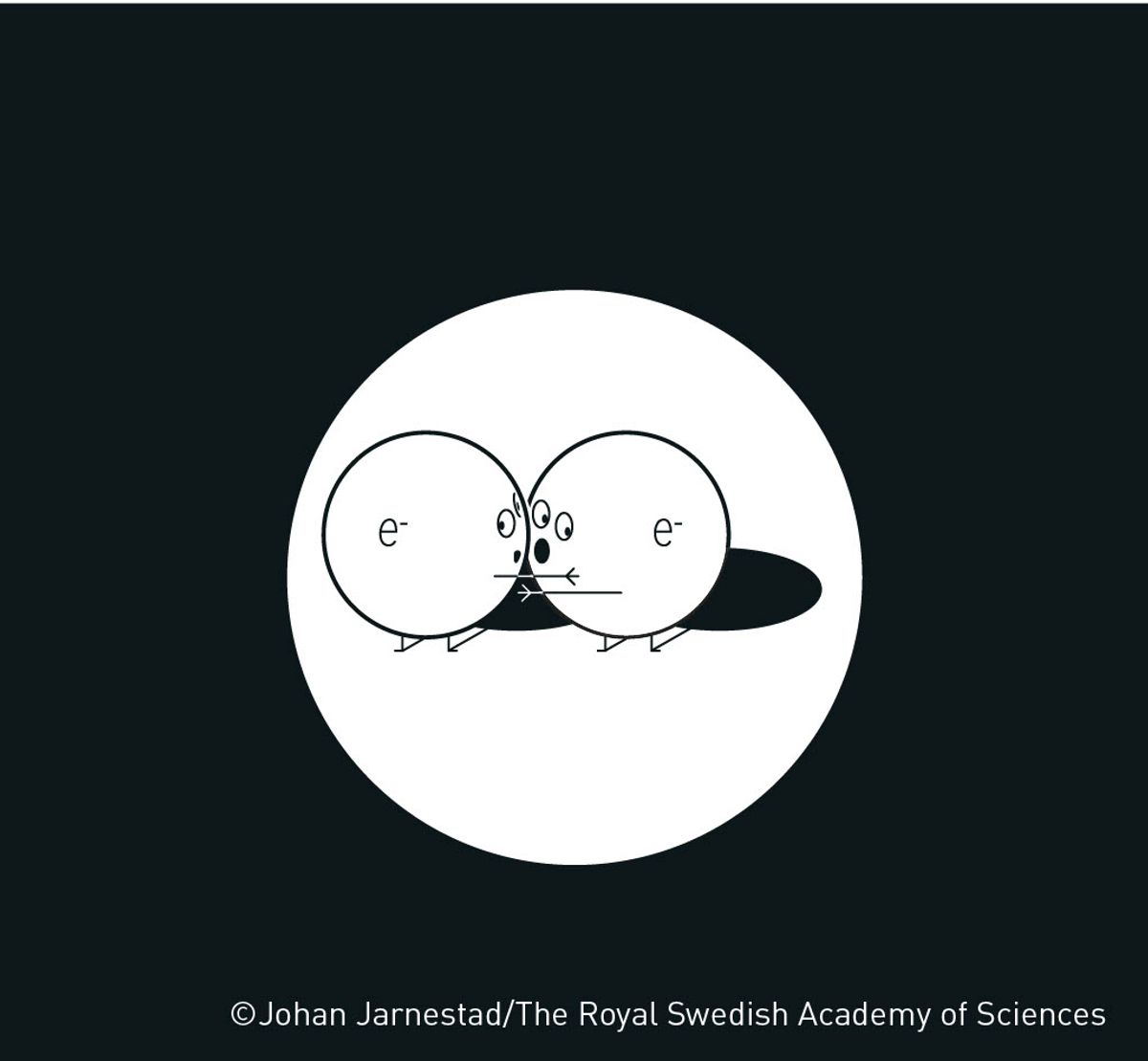
“The ability to generate attosecond pulses of light has opened the door on a tiny, extremely tiny, timescale, and it’s also opened the door to the world of electrons,” said Olsson. In 1925, theoretical physicist Werner Heisenberg opined that the world of electrons was impossible to capture. “Thanks to attosecond physics, this is now starting to change, and we are starting to explore this world,” said Olsson.
See also “Nobel Prize for mRNA Vaccines”
Electrons orchestrate matter from electronics to chemical reactions throughout the body. To investigate incredibly fast moving electrons, the physicists needed to develop specialized technology. Ordinary laser systems cannot generate anything shorter than a femtosecond burst of light.
In 1987, one year after she received her PhD, L’Huillier and her colleagues performed a groundbreaking experiment.1 They shone a powerful infrared laser light through a noble gas and measured the photons emerging from the interaction using a spectrometer. When the light interacted with the gaseous atoms, some electrons gained extra energy that was emitted as light.
When they analyzed the data, the physicists observed a number of overtones, or frequencies higher than the base frequency of the laser. The overtones exhibited a reduced intensity relative to the base intensity of the laser, with many overtones plateauing in intensity. This was the first time that physicists observed a clear demonstration of an overtone plateau. Over the next decade, L’Huillier used a variety of methods to dissect what generated the intensity plateau of the overtones, and investigated how she could exploit this feature.
Overtones interact with one another. When the peaks in the frequency coincide, this increases the intensity of the light creating a train of attosecond pulses. This is what occurs for a single atom, but many atoms must work together. “If each member of the orchestra would be playing whatever they want, the audience would just experience noise, but if they play in sync, and of course you have the conductor making them play in sync, then we get music,” Larsson explained during the press conference.
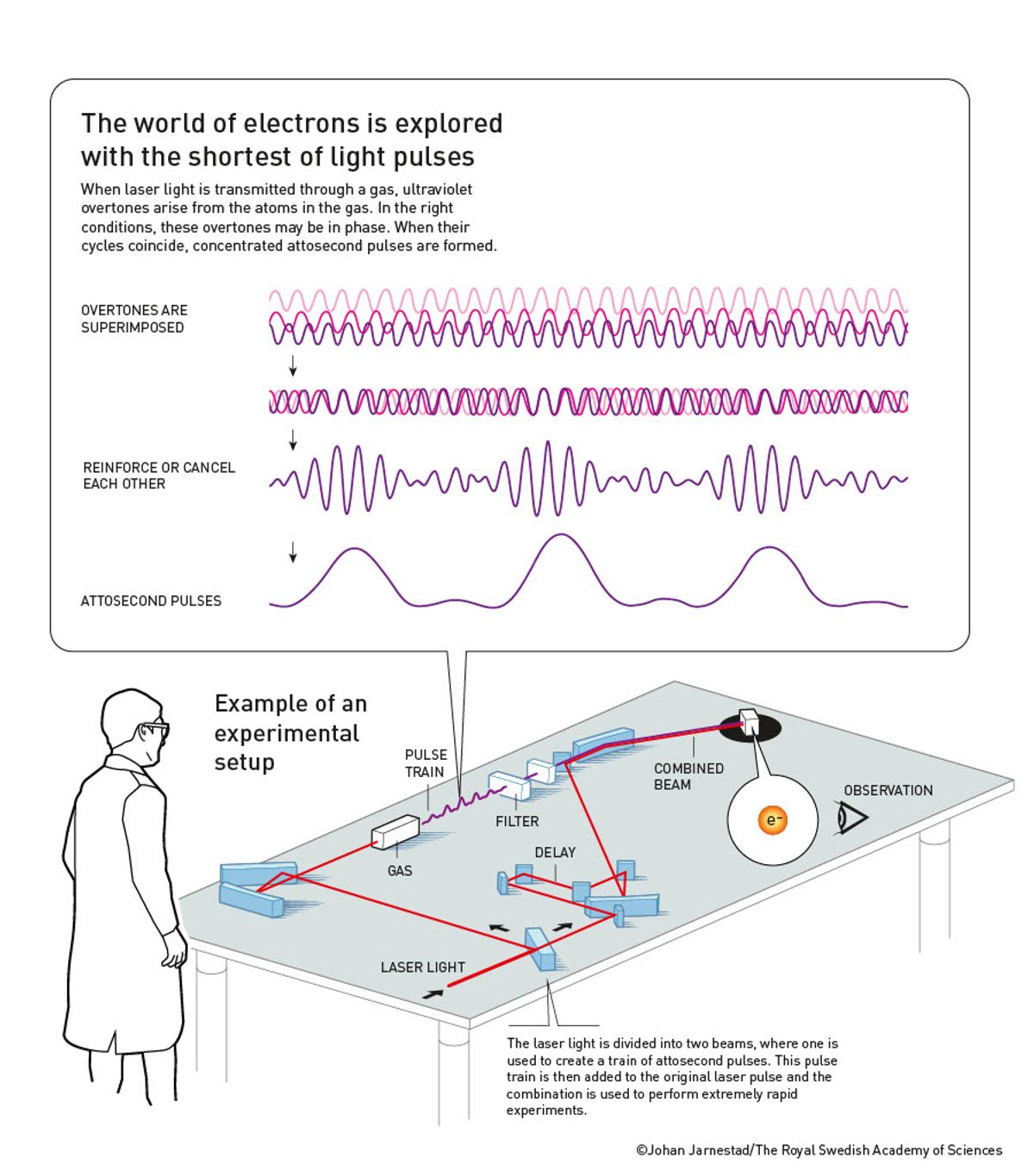
In the 1990s, physicists theorized how to conduct the overtones to get them to work in sync. Then in 2001, a breakthrough came in experimental physics when Agostini and Krausz independently generated two different methods for generating pulses of light on the attosecond timescale. Agostini’s method, Reconstruction of Attosecond Beating By Interference of Two-second Transitions (RABBIT), generated a pulse train of consecutive 250 attosecond pulses.2 Krausz’s method, the streaking technique, produced isolated 650 attosecond pulses.3 Physicists still use these two techniques, with some slight modifications, today for attosecond experiments.
The discoveries by L’Huillier, Agostini, and Krausz transformed fundamental research in attosecond physics, but also opened the door to future applications across chemistry, medicine, and electronics. For example, in attochemistry, scientists could control electrons on their own timescales using light in order to explore the behaviors of electrons in molecules.4 Short pulses generated in attosecond physics may also facilitate ultrafast switching from insulators to conductors, which could produce extremely fast electronics.
Krausz is exploring the use of attosecond physics for molecular fingerprinting with implications for medical diagnostics of biological samples.5 Exposing a blood sample to a short attosecond pulse excites thousands of molecules. When Krausz and his team analyzed light emitted from the dancing molecules in liquid biopsies with attosecond precision, they detected tiny changes in the spectral signatures in the blood.6 “By doing this, there is the hope in the future that you will be able to capture whether a person has developed, for example, lung cancer,” said Larsson in the press conference. “If you can diagnose cancer at the very early stage, treatment will be much more successful.”
L'Huillier is the fifth woman to receive the Nobel Prize for Physics. “This is the most prestigious prize, and I’m so happy to get this prize. It’s incredible. As you know, there are not so many women who get this prize so it’s very, very special,” said L’Huillier at the press conference.
References
- Ferray M, et al. Multiple-harmonic conversion of 1064 nm radiation in rare gases. J Phys B. 1988;21(3):L31-L35.
- Paul PM, et al. Observation of a train of attosecond pulses from high harmonic generation. Science. 2001;292(5522):1689-1692.
- Hentschel M, et al. Attosecond metrology. Nature. 2001;414:509-513.
- Merritt ICD, et al. Attochemistry: Is controlling electrons the future of photochemistry? J Phys Chem Lett. 2021;12(34):8404-8415.
- Pupeza I, et al. Field-resolved infrared spectroscopy of biological systems. Nature. 2020;577:52-59.
- Huber M, et al. Infrared molecular fingerprinting of blood-based liquid biopsies for the detection of cancer. eLife. 2021;10:e68758.