ABOVE: © ISTOCK.COM, Dr_Microbe
Sitting on her dirt throne and swollen with eggs, a termite queen is tended to by her sterile subjects. All of the insects in the colony are specialists in what they do: Workers forage and construct the mound, while soldiers defend it from invaders. Division of labor among social insects maximizes efficiency to ensure that the royal genes make it to the next generation. Yet, teeming in the soil around them, a similar scenario plays out in a whole other domain of life.
The evolution of bacteria has a lot in common with higher eukaryotes such as birds and bees, said Ákos Kovács, a microbiologist at the Technical University of Denmark. Studying single-celled organisms may help scientists better understand multicellular life, he added.
Kovács’s team investigates “sociomicrobiology,” an emerging field that examines the rich social lives of microbes. In contrast to the free-living, or planktonic, bacteria commonly studied in the lab, most prokaryotes in nature settle down in complex communities, often consisting of several interacting species. These biofilms of aggregating microbes are everywhere: on rocks, bathtubs, and medical devices. In fact, you’re supporting a heap of these communities right now. The slimy coating on unbrushed teeth is biofilm, and the beneficial microbes on your skin and gut often grow collectively.
They were killing each other like crazy.
—Bonnie Bassler, Princeton University
Biofilms form when groups of bacteria cover themselves in a sticky mixture of sugars, protein, and DNA. This extracellular matrix glues bacteria to surfaces and serves as a slimy shield, protecting cells in the interior from predators and antibiotics. Conventional drugs become ensnared in the matrix, tangled in a molecular mesh that prevents them from penetrating the biofilm’s inner layers. What’s more, oxygen depletion causes cells in the center to enter a hibernation-like state, making them tolerant to antibiotics that target metabolic processes. In fact, biofilms can withstand doses up to 1,000 times greater than their planktonic counterparts.
See: “Inside Versus Out: A New Form of Bacterial Cooperation”
Within the biofilm, bacteria take on different responsibilities. Some individuals focus on reproduction to expand the colony, while others specialize in construction, oozing polysaccharides and proteins that make up the extracellular matrix. And other bacteria defend the community, building molecular weapons that shoot competitors with toxins.
These tasks aren’t fixed for life. As the biofilm matures and the community needs change, an individual bacterium may take on new responsibilities. Kovács’s team has found that in populations of the soil bacterium Bacillus subtilis, most microbes assume responsibility for matrix production during early development when they are little more than a throng of unconnected cells. But once construction progresses, some cells will switch to producing spores or useful enzymes.1
A microbial metropolis
So how do bacteria dole out chores? It’s partly stochastic, said Kovács. By amplifying random fluctuations in cellular reactions, individual bacteria specialize into distinct roles. For instance, the cells within a B. subtilis biofilm that are engaged in protease production are randomly determined.
Task delegation can also be influenced by a bacterium’s location within the biofilm, said Daniel Dar, a researcher of microbial systems at the Weizmann Institute of Sciences. Just as different boroughs of a city might differ in levels of air pollution or abundance of organic supermarkets, different parts of the biofilm are exposed to wildly different levels of oxygen and nutrients. Bacteria sense these microenvironments and adapt by up or down regulating the expression of certain genes to influence different cell processes.
As a postdoctoral researcher in Dianne Newman’s lab at the California Institute of Technology, Dar developed a way to track gene activity across the entire biofilm with submicrometer resolution. He used a tool known as parallel sequential fluorescence in situ hybridization (par-seqFISH) on populations of Pseudomonas aeruginosa, a species often found in the lungs of people with cystic fibrosis.
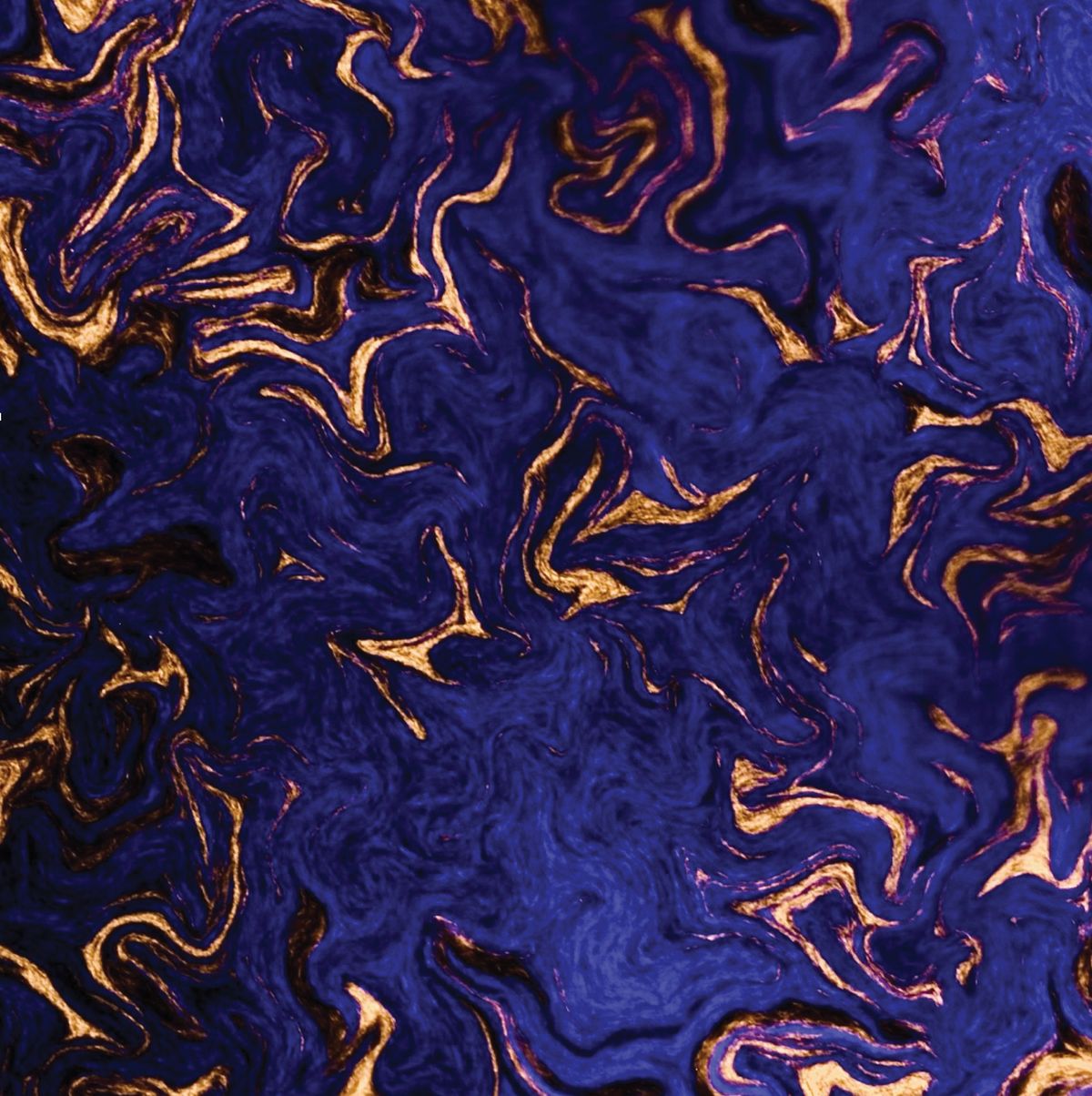
Rather than detecting each gene with a single fluorophore as with standard FISH, sequential FISH targets multiple probes to different regions of a transcript, then decodes its identity based on the color sequence. Dar performed the technique on several stages of bacterial growth—from planktonic to mature biofilms—in a single experiment.
By lighting up expression of more than 100 genes, the researchers uncovered what looked like a color-by-numbers map of zoning regulations. Different clusters of bacteria showed diverse patterns of gene activity corresponding to altered metabolic states depending on their locations within the biofilm. For instance, microbes in the bottom portion of the mature biofilm had activated a set of genes that code for digestive enzymes, while their westerly neighbors prioritized transcription of genes involved in defense.2
One such defense mechanism was the enhanced production of pyocin, a toxin that affects other bacterial species, by Pseudomonas aeruginosa as they begin to aggregate. As the biofilm forms, clusters of cells synthesize pyocin and release it by annihilating themselves. The bacteria burst, sprinkling the poison and DNA into their surroundings. This seemingly suicidal behavior gives the biofilm the best chance of survival: The toxin kills competitors, while the DNA binds to molecules within the matrix, strengthening its structure and accelerating biofilm growth.
While the tool could provide new leads for treating biofilm infections, researchers also need to consider environmental factors. “It’s difficult to understand behavior without understanding context,” said Newman, who teams up with chemists to visualize metabolite levels. One way she hopes to do this is by tagging oxygen molecules to illuminate the gradients between the oxygenated extremities and the anoxic inner core. Combined with spatial transcriptomic techniques and other methods, the approach could allow researchers to learn how conditions within the biofilm determine bacterial behavior.
The Biofilm Life CycleBacterial communities form when free swimming bacteria reversibly attach to a surface. At high cell densities, many species trigger processes involved in biofilm formation, including the production of extracellular matrix. The matrix adheres the community to the surface and provides protection from predators and antibiotics. As the biofilm matures, bacteria specialize to perform different tasks within the biofilm. For instance, a subset of cells sprouts flagella and swims off from the community to colonize new surfaces. ![]() modified from © istock.com, Olha Pohrebniak |
Chromosomal contortions
Division of labor doesn’t only arise from differences in gene expression but can also be triggered by irreversible genetic changes. Among Streptomyces coelicolor, a soil-dwelling bacteria that forms fungal-like structures, deletions of large chunks of their chromosome causes a subset of cells to specialize in antibiotic synthesis. More than half of the antibiotics used to treat human infections are produced by S. coelicolor.3
Although the colonies start out as clones, unstable DNA regions quickly acquire mutations. In a paper published this year in Molecular Systems Biology, scientists reported how the S. coelicolor genome is split into two main sections. The more stable end of the chromosome houses genes that synthesize antibiotics, while the growth-promoting genes are located on the more fragile end. Mutations in these delicate regions often erase sequences associated with growth and replication, generating strains that are specialized in antibiotic secretion.4
Specialization comes at a cost, however. Like sterile worker insects, antibiotic producers are unlikely to reproduce, yet the whole colony benefits from their labor. In some cases, mutants were up to 10,000 times less likely to release spores.
Other work revealed how genetic diversity can have more insidious causes. In a paper published last year in Cell, researchers described how microbes shoot their compatriots with toxins to drive the emergence of useful mutations.5
To defend themselves from competitors, Gram-negative bacteria assemble a molecular machine gun called the type VI secretion system (T6SS). Shaped like a spear, the weapon is loaded with toxins that are fired into adversaries by puncturing their cell walls. To avoid poisoning themselves, the bacteria synthesize immunity proteins that neutralize the toxin.
See: “How Commensal Gut Bacteria Keep Pathogens in Check”
Scientists have known for decades that bacteria use the T6SS to snuff out their non-kin neighbors, or strangers that are invading their territory. But no one imagined that it’s used to kill their siblings, said Bonnie Bassler, a Princeton University molecular biologist and an early pioneer of research on bacterial communication.
The study explained something that researchers call sectoring, which occurs when a biofilm partitions into zones made up of genetic variants, despite the bacteria starting out as clones. Bassler’s team grew biofilms made up of the cholera-causing bacteria Vibrio cholerae. Just before the community segregated into mutants, cells within the biofilm started shooting their neighbors with toxins. “They were killing each other like crazy,” said Bassler.
To save energy, bacteria only start to assemble their molecular weapons when there’s plenty of other microbes around. It’s controlled by a process called quorum sensing, a form of bacterial communication. Each bacterium leaks a chemical trickle, which increases in concentration as the population grows. Once a threshold is reached, bacteria activate quorum-sensing genes in unison, triggering group behaviors such as biofilm formation.
The microbial massacre may drive the emergence of quorum-sensing mutants that can resist killing, such as variants that synthesize more of the polysaccharide that can serve as a protective armor. Some of the mutations that Bassler’s team spotted in V. cholerae have also been found in clinical samples, suggesting that kin killing could be responsible. Murdering your siblings might be a way of speeding up evolution, said Bassler. Bacteria are mutating in all sorts of ways, but “only mutants that can resist T6SS killing survive and replicate, so they flourish,” she added.
Killing the CoreSpatial transcriptomics could provide clues on how to sneak antibiotics into the biofilm’s impenetrable core by identifying proteins essential for biofilm formation. Dar’s par-seqFISH analysis pointed to a group of metabolites known as phenozines, which are expressed as the P. aeruginosa begin to clump together. The finding supports previous data from Dianne Newman’s group on how pyocyanin, a type of phenozine, encouraged bacterial cells to interact. Destroying pyocyanin using the enzyme PodA made biofilms more vulnerable to antibiotics, but PodA is unstable and synthesized naturally in minute quantities.9 Newman teamed up with Sarel Fleishman to figure out a way to tweak PodA’s structure. A structural biologist at the Weizmann Institute of Sciences, Fleishman used a computational approach to predict mutations that increase the protein’s stability. When Newman’s group applied the customized enzyme to populations of P. aeruginosa, the antibiotic destroyed a larger proportion of the biofilm than did the drug on its own.10 ![]() E. coli biofilms observed under a Mesolens reveal channel structures permeating throughout the colony. Liam Rooney, University of Strathclyde Advances in optical microscopy have provided other clues to weakening the biofilm’s core. By redesigning the objective lens, Gail McConnell, a physicist at the University of Strathclyde in the United Kingdom developed a tool to image embryos with high resolution and magnification in a single shot. When Liam Rooney joined McConnell’s lab, he was the first microbiologist to get his hands on it and to glimpse the inner workings of a microbial city. Before Rooney began the project, he had never seen a high-resolution image of the interior of a biofilm. “We assumed it was just a ball of homogenous cells, all densely packed with no real internal structure,” said Rooney. By applying the tool known as the Mesolens to Escherichia coli biofilms, the team observed a network of microscopic water channels meandering through the colony’s interior.11 These conduits may help the biofilm traffic nutrients, oxygen, and other resources into the starving core, just as our blood vessels ferry molecules around our body. The channels could be exploited to maneuver antibiotics into the heart of the biofilm, said Rooney. |
Worker’s strike
By understanding how division of labor is controlled, researchers might be able to disrupt this process to spark societal collapse. Initial findings have pointed to electricity to do just that.
Electricity can kill bacteria, but lower doses can also stimulate growth of specific cell types, said Gürol Süel, a biophysicist at the University of California, San Diego. Over the past decade, Süel’s team has uncovered how bacteria communicate through electrical impulses, much like a giant action potential that passes through the entire biofilm. Electrical signaling allows cells within the biofilm to regulate growth, share nutrients and recruit outsiders to join the community.
In his new work, Süel’s team focused on two major biofilm cell types: motile cells, which swim off and colonize new surfaces, and matrix-secreting cells, which churn out the sticky polymers that glue the rest of the colony together.
The team developed a device to electrically stimulate a growing B. subtilis biofilm. Despite being genetically identical and exposed to the same microenvironment, the weak electric shock promoted growth of motile cells but not matrix producers. Electrical stimulation skews the ratio of cell types within the community, which is soon overrun with swimmers.6
This could lead to biofilm dispersal since less matrix is produced to hold the community together, the researchers suggested. Since the matrix also blocks antibiotics from the biofilm’s core, it could make them more vulnerable to treatment, they added. Dispersing bacterial communities by switching their responsibilities is something that Süel’s group is currently pursuing.
Another promising strategy is to target the shared substances, digestive enzymes, matrix polymers and secretion systems, that allow microbial societies to thrive, said Kovacs. When conventional antibiotics are applied to biofilms, resistant cells soon have the upper hand and take over the population. But when bacteria are exposed to biofilm-dispersing drugs that inhibit production of “public goods,” resistant cells are at the mercy of their nonresistant counterparts. The new resources are shared and exploited by surrounding cells, which benefit without expending any energy. The nonresistant cells prevail, growing until the public good runs out and the biofilm disperses.
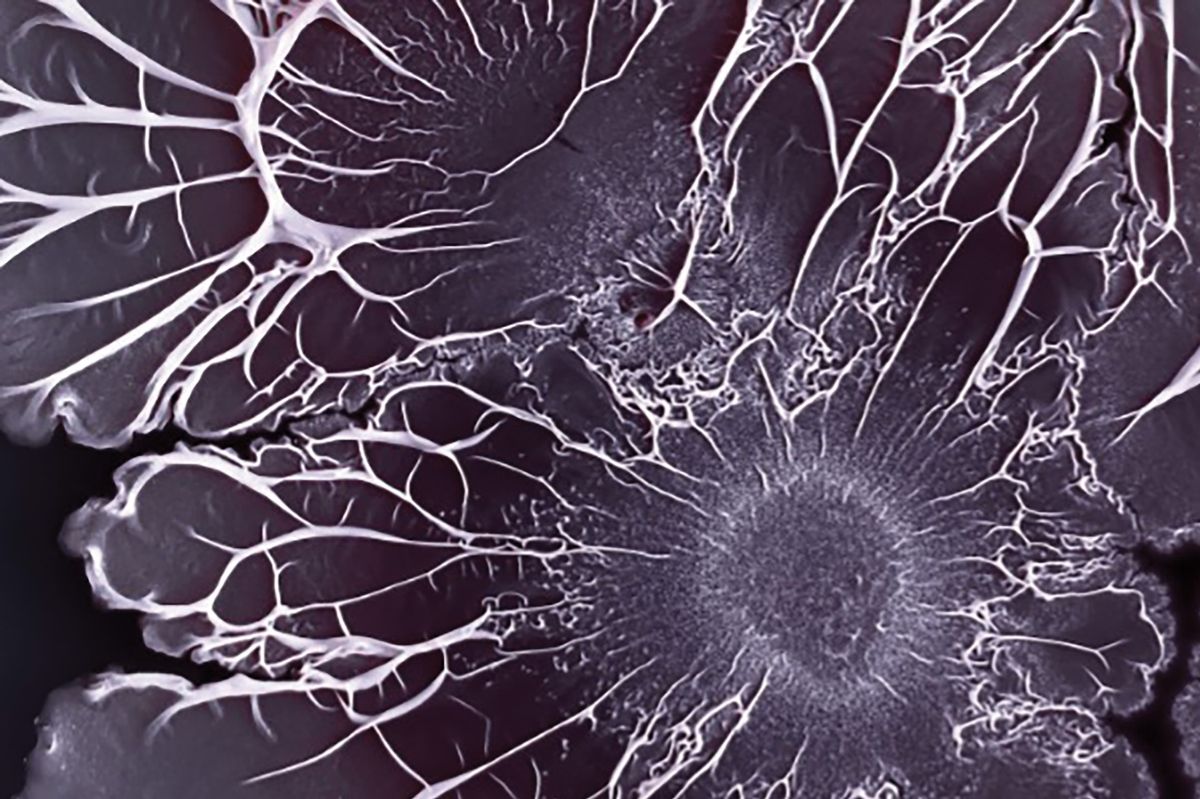
Testing this approach experimentally, microbiologist Hans Steenackers and colleagues at KU Leuven in Belgium grew Salmonella incapable of synthesizing matrix polymers. Unlike wild type microbes, the mutant bacteria were less likely to form biofilms and more vulnerable to antibiotics. No cells evolved resistance over the 40-day experiment, while resistance emerged against standard antibiotics in a matter of days. When the team hunted down some resistant strains and added them to the Petri dish, the nonresistant cells quickly outcompeted their neighbors.7
Interfering with public behaviors has potential beyond microbiology, Steenackers wrote in a review on the topic.8 The strategy could be extended to treating cancer, which grows in a matrix-like microenvironment, or to disperse insect swarms. Insects communicate via pheromones in a way similar to how bacteria communicate via quorum sensing, said Steenackers, and disrupting cooperation may be a more sustainable approach than typical insecticides.
Studying bacterial cooperation is not only key for targeting pathogenic biofilms, but it could also shed light on multicellular life, said Kovács.
Spatial transcriptomics could provide clues on how to sneak antibiotics into the biofilm’s impenetrable core by identifying proteins essential for biofilm formation. Dar’s par-seqFISH analysis pointed to a group of metabolites known as phenozines, which are expressed as the P. aeruginosa begin to clump together. The finding supports previous data from Dianne Newman’s group on how pyocyanin, a type of phenozine, encouraged bacterial cells to interact. Destroying pyocyanin using the enzyme PodA made biofilms more vulnerable to antibiotics, but PodA is unstable and synthesized naturally in minute quantities.
Newman teamed up with Sarel Fleishman to figure out a way to tweak PodA’s structure. A structural biologist at the Weizmann Institute of Sciences, Fleishman used a computational approach to predict mutations that increase the protein’s stability. When Newman’s group applied the customized enzyme to populations of P. aeruginosa, the antibiotic destroyed a larger proportion of the biofilm than did the drug on its own.
Advances in optical microscopy have provided other clues to weakening the biofilm’s core. By redesigning the objective lens, Gail McConnell, a physicist at the University of Strathclyde in the United Kingdom developed a tool to image embryos with high resolution and magnification in a single shot. When Liam Rooney joined McConnell’s lab, he was the first microbiologist to get his hands on it and to give us glimpse into the inner workings of a microbial city.
Before Rooney began the project, he had never seen a high-resolution image of the interior of a biofilm. “We assumed it was just a ball of homogenous cells, all densely packed with no real internal structure,” said Rooney.
By applying the tool known as the Mesolens to Escherichia coli biofilms, the team observed a network of microscopic water channels meandering through the colony’s interior. These conduits may help the biofilm traffic nutrients, oxygen, and other resources into the starving core, just as our blood vessels ferry molecules around our body. The channels could be exploited to maneuver antibiotics into the heart of the biofilm, said Rooney.
References
- Otto SB, et al. Privatization of biofilm matrix in structurally heterogeneous biofilms. mSystems. 2020; 5(4): e00425-20.
- Dar D, et al.. Spatial transcriptomics of planktonic and sessile bacterial populations at single cell resolution. Science. 2021; 373(6556).
- Zhang Z, et al. antibiotic production in Streptomyces is organized by a division of labor through terminal genomic differentiation. Sci Adv. 2020; 6(3): eaay5781.
- Colizzi ER, et al. Evoltiion of genome fragility enables microbial divisions of labor. Mol Syst Biol. 2023; 19(3): e11353.
- Mashruwala AA, et al. Quorum-sensing- and type VI secretion-mediated spatiotemporal cell death drives genetic diversity in Vibrio cholerae. Cell. 2022; 185(21):3988-3979.
- Comerci CJ, et al. Localized electrical stimulation triggers cell-type specific proliferations in biofilms. Cell Syst. 2022; 13(6):488-498.
- Dieltjens L, et al. Inhibiting bacterial cooperation is an evolutionarily robust anti-biofilm strategy. Nat Comms. 2020; 11(107).
- Lissens M, et al. Evolution-proof inhibitors of public good cooperation: a screening strategy inspired by social evolution theory. FEMS Microbiol Rev. 2022; 46(5):1-18.
- Costa KC, et al. Pyocyanin degradation by a tautomerizing demethylase inhibits Pseudomonas aeruginosa biofilms. Science. 2016; 355(6321):170-173.
- VanDrisse CM, et al. Computationally designed pyocyanin demethylase acts synergistically with tobramycin to kill recalcitrant Pseudomonas aeruginosa biofilms. Proc Natl Acad Sci USA. 2021; 118(12).
- Rooney LM, et al. Intra-colony channels in E. coli function as a nutrient uptake system. ISME J. 2020; 14(10):2461-2473.