Today, PCR is ubiquitous in molecular biology laboratories. As one of the first experiments that young scientists master, it is easy to take for granted. But developing this research lab staple required a team of scientists painstakingly repeating trials and troubleshooting parameters to finally amplify a single, small, targeted DNA sequence.
“Once you’ve shown that it works, this idea is kind of simple and almost seems, in retrospect, obvious,” said Henry Erlich, currently a senior scientist at the Children’s Hospital Oakland Research Institute. “But the idea that you could amplify a specific targeted DNA sequence using an oligonucleotide primer and DNA polymerase was not at all obvious.” Erlich led the team of scientists at the Cetus Corporation that developed the PCR method.
In the 1950s, scientists discovered Escherichia coli DNA polymerase and immediately put it to use synthesizing DNA molecules for many research purposes. By adding a complementary oligonucleotide primer, scientists could control where DNA synthesis began, but not where it ended. Complex sequences such as those in the human genome contained multiple places where a single primer could hybridize, leading to a complete lack of specificity in the end products. Kary Mullis, a chemist in the department of human genetics at Cetus Corporation, decided to solve the primer specificity problem.

During a night-time drive along the California coast in 1983, an idea that would revolutionize molecular biology struck Mullis. By building upon the simple DNA synthesis and sequencing techniques already in use, Mullis realized that he could achieve targeted DNA synthesis by adding a second primer to hybridize with the end of a desired sequence. As legend has it, further into the drive, Mullis realized that performing multiple cycles of the polymerase reaction with two primers would exponentially amplify the target sequence.1
Mullis eagerly took his novel idea to the lab and recruited his research assistant, Fred Faloona, to attempt the reaction. After combining the necessary components into a test tube— template DNA, nucleotides, primers, and polymerase—they were disappointed to observe a large smear after performing gel electrophoresis on the completed reaction. Mullis was convinced that his method worked, but other scientists were highly skeptical.
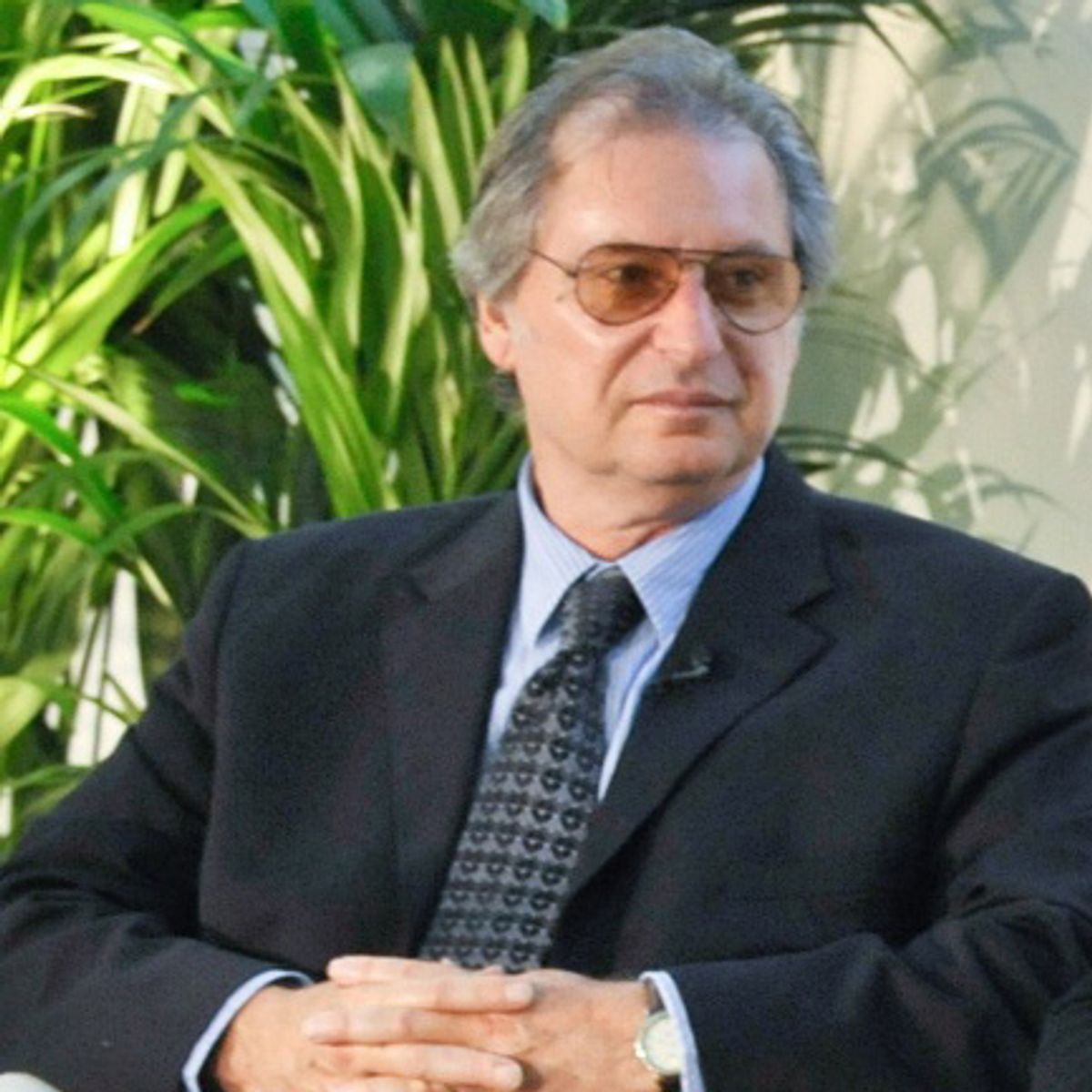
Tom White, then head of research at Cetus Corporation, saw potential in Mullis’ idea and asked Erlich and the human genetics team he led, including Randy Saiki, Steve Scharf, and Norm Arnheim, to work in parallel with Mullis. The team attempted to amplify the same fragment that Mullis targeted—a 110 base pair segment from the human β-globin gene. At first, they could not determine if they achieved specific target amplification; their gel electrophoresis results were also large smears. They needed to determine if their product was part of this smear.
Over time, the team experienced a series of minor celebrations as they inched closer to the PCR method we are familiar with today. A crucial moment occurred toward the end of 1984 when Erlich and Saiki performed a Southern blot on their smeared DNA sample using a radioactive probe complementary to the β-globin fragment. Erlich and his team were extremely excited by the result; the blot showed a distinct radioactive band at 110 bp. “We thought, ‘Oh my god, this thing could actually work,’” Erlich recalled.
The team next tackled how to improve the reaction’s efficiency. “Only about 1 percent of what was synthesized in that tube was β-globin,” said Erlich.
From the beginning, the Klenow polymerase the scientists were using was problematic. It degraded at 94°C, the temperature required to denature the DNA target; this meant that the scientists had to open the PCR reaction tube at the start of every cycle to add fresh enzyme. Additionally, Klenow worked best at 37°C, a temperature that was too low for the primers to bind precisely to their complementary sequences. “The PCR primers hybridized to various places in the genome and that was the reason that the system wasn’t operating as diagrammed on a blackboard. We were getting nonspecific priming, and therefore we were synthesizing a lot of DNA that was not the target,” explained Erlich.
The idea that you could amplify a specific targeted DNA sequence using an oligonucleotide primer and DNA polymerase was not at all obvious.
—Henry Erlich, Children’s Hospital Oakland Research Institute
Still dedicated to making the technique work, Cetus Corporation scientists David Gelfand and Susanne Stoffel purified a thermostable DNA polymerase from Thermus aquaticus—the now-famous Taq polymerase—which survives denaturing temperatures and copies DNA at 70°C.2 “We no longer had to add enzyme at every step,” said Erlich. “That was actually a major breakthrough in the development of PCR technology—that you could just put everything in. You didn’t have to keep adding enzyme and opening the tube. You could just put everything in the tube, close it, and cycle the temperature, and PCR would occur.”
Additionally, raising the annealing and elongation temperatures increased primer binding specificity. “With this thermostable DNA polymerase, if you just ran a gel with the whole reaction, you’d see a discrete band at the expected position in the gel for a 110 base pair fragment,” Erlich explained. “If you used a [detection] probe, that was clearly β-globin.”
At this stage, researchers changed the reaction temperature by manually moving tubes between water baths set at different temperatures, a procedure that was time consuming and not sustainable long term. Cetus Corporation engineers built a crude thermocycler with protruding tubes and piping to change the temperature in the PCR vessel. “We called it Mr. Cycle, after the then popular Mr. Coffee,” Erlich remembered. After the addition of Taq to the protocol, Mr. Cycle needed an upgrade to progress more quickly through the reaction temperatures. Thanks to a joint venture with the Perkin Elmer Corporation in 1985, the first modern PCR thermocyclers were born.
With an automated PCR reaction that faithfully amplified target DNA now consistently working, the Cetus Corporation scientists quickly applied the technology to many diverse projects. Almost immediately, they added restriction sites to the primers to facilitate cloning reactions. Russ Higuchi developed quantitative PCR and John Sninsky quickly adapted the technique to detect HIV viral loads, which was instrumental during the AIDS epidemic. PCR was also a game changer for Erlich’s own work characterizing individuals’ polymorphic HLA genes. This led to PCR’s first appearance in the courtroom in a criminal case where Erlich reported detecting HLA in degraded DNA samples. A year later, he used PCR for the first time in a postconviction exoneration. “The idea that some basic research that I had been doing could be used in the area of criminal justice and have a practical and significant effect was very, very exciting,” said Erlich. “To be honest, we all thought this was going to be a big deal. But I think it’s turned out over the last 35 years to be an even bigger deal than we imagined.”
References
- K.B Mullis, “The unusual origin of the Polymerase Chain Reaction,” Scientific American. 262:56-65, 1990.
- R.K. Saiki et al., “Primer-directed enzymatic amplification of DNA with a thermostable DNA polymerase,” Science, 239:487-91, 1988.