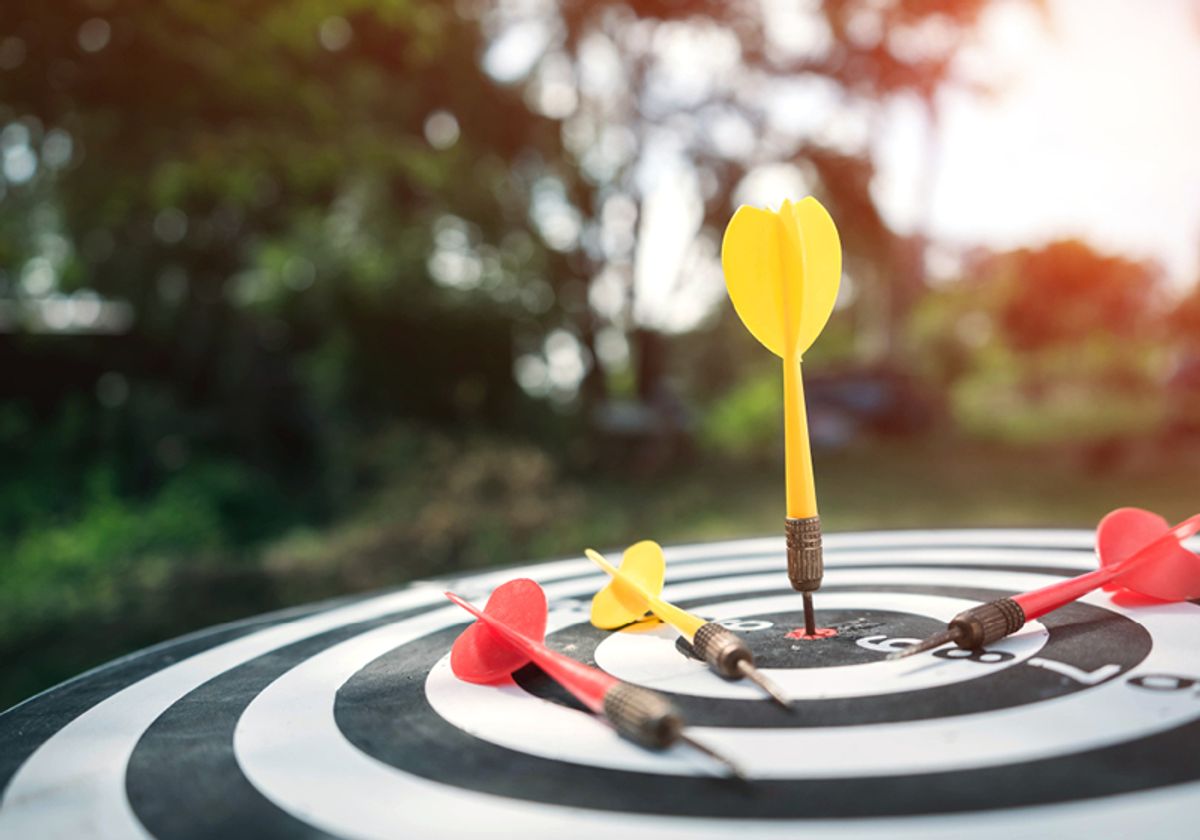
Genome editing with CRISPR technologies has progressed rapidly, from foundational biology research to clinical applications. As Cas9-based gene therapies for diseases such as sickle cell anemia travel the road to FDA approval,1 many scientists search for techniques that take genome engineering to the next level. Specifically, researchers are turning their attention to tools for editing RNA instead of DNA, which may yield more precise gene expression control and enable direct targeting of noncoding DNA transcripts.2
Conventional CRISPR-Cas9 techniques rely on the Cas9 nuclease and a guide RNA (gRNA) component, which directs the nuclease to cut complementary DNA sites. Some potential roadblocks to this technique include off-target nuclease interactions with non-target DNA, and limited editing at desired target regions.1 Scientists perform large-scale screens for on-target and off-target sites via next generation sequencing, which helps them identify more precise and effective CRISPR technologies.3
“Ten years ago, it was super hard to think about targeting individual genes. And now that’s something, with these kinds of CRISPR screens, we can do routinely,” said Neville Sanjana, a geneticist at New York University, whose laboratory develops technologies to dissect the underpinnings of disease genetics. Recently, Sanjana’s research team investigated the potential of the Cas13 nuclease, which is the RNA-editing cousin of Cas9.
Ten years ago, it was super hard to think about targeting individual genes. And now that’s something, with these kinds of CRISPR screens, we can do routinely.
- Neville Sanjana, New York University
In work published in Nature Biotechnology, Sanjana and his collaborator David Knowles from Columbia University paired the power of CRISPR screening and deep learning to identify the rules governing Cas13-mediated transcriptome engineering.4 The researchers designed and tested roughly 200,000 Cas13 gRNAs that targeted essential genes in human cells. They examined how different types of gRNA mutations affected editing and they created a large-scale dataset to train a convolutional neural network, which they named targeted inhibition of gene expression via gRNA design (TIGER). The researchers used TIGER to predict Cas13 gRNA efficacy and precision.
TIGER revealed which factors scientists need to consider when developing tightly controlled RNA-editing systems, such as the types of target/gRNA mismatches that have the greatest influence on Cas13 activity. These findings open the door to the next frontier of CRISPR strategies, allowing researchers to modulate the dose of editing, rather than using gene editing as a binary on/off switch.
“Modulating the gene function by 20 percent versus 50 percent versus 100 percent, in a lot of cases, can give you different results. But one major bottleneck is that, right now, we don't have the precise tool to exactly control the gene expression reduction,” said Wei Li, a computational biologist from George Washington University and Children’s National Hospital, who was not involved in the study. “If you think about adjusting the volume on your speakers, in most cases, you want to precisely adjust it such that you make yourself most comfortable with the volume. This is exactly what they did; I think it's really exciting.”
Sanjana’s big picture goal for TIGER and Cas13 looks beyond gene therapies to answer a fundamental question, how does the genome work? Towards this end, Cas13 provides an additional level of control that Cas9 technologies inherently lack; RNA editing allows researchers to directly modify noncoding RNAs (ncRNAs).2,3
For scientists seeking to unlock the secrets of the genome, ncRNA transcripts are a large piece of the puzzle with potentially critical roles in diverse biological and disease processes.2 Although this puzzle remains incomplete, Sanjana hopes to fill in the gaps using Cas13 technology. “As we were developing these CRISPR screens to target all genes in the genome, I always felt like, well, what about those transcripts,” he said. “Wouldn't it be great if we could do the same thing, take advantage of the programmability of CRISPR, which is very similar between Cas13 and Cas9, and use it to also do these kinds of pooled screens where we could target not thousands of genes, but thousands of noncoding RNAs, to really understand that part of the genome.”
References
- Kingwell K. First CRISPR therapy seeks landmark approval. Nat Rev Drug Discov. 2023;22:339-341.
- Sun YM, Chen YQ. Principles and innovative technologies for decrypting noncoding RNAs: from discovery and functional prediction to clinical application. J Hematol Oncol. 2020;13(1):109.
- Cheng X, Li Z, et al. Modeling CRISPR-Cas13d on-target and off-target effects using machine learning approaches. Nat Commun. 2023;14(1):752.
- Wessels HH, et al. Prediction of on-target and off-target activity of CRISPR-Cas13d guide RNAs using deep learning. Nat Biotechnol. [published online: July 03, 2023].