© GETTY IMAGES/STEPHEN ASHTON/EYEEMDown a dirt path outside of the village of Meliandou in Guinea once stood a tall, hollow tree where children used to play. Not anymore. This tree, now notorious as the potential starting point of the deadly Ebola outbreak that ripped through West Africa a few years ago, was burned after the disease sickened and killed hundreds of people over a four-month period. More than 10,000 ultimately succumbed to the disease between 2014 and 2016.
In April 2014, just a few months after the outbreak began, epidemiologist Fabian Leendertz of the Robert Koch Institute in Berlin and his colleagues went on a month-long expedition in southeastern Guinea to identify the source of the epidemic, which was suspected to have jumped from animals to humans. Fairly quickly, the team ruled out apes and other large animals as the zoonotic host for this Ebola outbreak. Numbers of...
Another possibility that Leendertz and his team considered was bats. Local children commonly hunted the flying mammals, the scientists learned. In time, Meliandou villagers told Leendertz and his colleagues about the tree. Children had been seen catching bats in its hollow trunk. The villagers led the scientists down the dusty path to show them what was left of it, describing how the sky “rained” bats as the tree became engulfed in flames. Sure enough, when Leendertz’s team collected soil samples from below the tree’s charred trunk, the researchers recovered DNA that belonged to Mops condylurus, an insectivorous bat species.1
The evidence seemed to point to bats as the source of Ebola, Leendertz says. Emile Ouamouno, a two-year-old considered to have been patient zero in the outbreak, died a few days after contracting a fever, and probably got infected with the virus while playing with bats in the tree, he adds, “but we have no scientific proof.” Indeed, the ultimate source of the outbreak remains a mystery—as is the case for many so-called zoonotic diseases.
Bacteria cause more zoonoses than any other pathogen type.
More than 6 out of 10 known infectious diseases and 3 out of 4 new or emerging infectious diseases are spread to humans from other animals, including livestock and wildlife, according to estimates published by the US Centers for Disease Control and Prevention (CDC). Public health officials and scientists from many disciplines have been working together to understand how, when, where, and why pathogens spill over into humans, in hopes of preventing future epidemics. Key to these efforts are surveillance programs that aim to spot outbreaks early and respond quickly. Scientists are also scouring wildlife populations to identify undiscovered pathogens that could cause future zoonotic disease outbreaks.
Other researchers are working to identify biological factors that affect the probability of interspecies pathogen leaps, such as the immunological response of infected animals and the pathways of human exposure, efforts that could help scientists predict when and possibly where future zoonotic outbreaks might occur. By mapping the distribution of mammal species that host zoonotic pathogens, for example, disease ecologist Barbara Han of the Cary Institute of Ecosystem Studies in Millbrook, New York, and her colleagues have been working to identify regional hotspots at the greatest risk for spillover. “Everyone wants to know how well we can do in terms of prediction with zoonotic diseases, probably more so now after Ebola and Zika,” Han tells The Scientist.
But even with a growing understanding of how species leaps happen, along with new data pointing to regions where spillover might occur, precise outbreak predictions aren’t guaranteed. Some scientists say that such pinpoint prediction isn’t even possible.
Searching for reservoirs
When hunting for the source of a zoonotic disease outbreak, scientists first work to identify the species that harbor that particular pathogen. These species are called reservoirs. Half of all carnivores are reservoirs of zoonotic disease, as well as some 20 percent of nonhuman primates and roughly 10 percent of rodent and bat species.
For many zoonotic pathogens, the main nonhuman reservoirs are relatively well known: dogs, foxes, bats, and cats for rabies; rodents for Lassa and hantaviruses; bats for Hendra and Marburg virus; and ticks, sheep, deer, rodents, and other small mammals for Borrelia burgdorferi, the bacterium that causes Lyme disease. For other pathogens, however, identifying the reservoirs has been more challenging, Leendertz says. And if scientists can’t find them, it’s hard to devise public health policies to prevent human infection.
EVER-PRESENT THREAT: Zoonotic pathogens come in diverse forms, from viruses such as Zika (left) to bacteria such as Salmonella (bottom right) to fungi, protozoa, and helminths. Such pathogens can lie in wait in reservoir species, including wildlife, livestock, and domestic animals such as dogs.ZIKA VIRUS: CDC GLOBAL/FLICKR; SALMONELLA: WIKIMEDIA COMMONS/ROCKY MOUNTAIN LABORATORIES, NIAID, NIH; PIG: © ISTOCK.COM/NEILYIMAGERY; DOG: © ISTOCK.COM/PEKIC
There have been hints that Ebola virus lurks in populations of large African wildlife, with an ongoing epidemic possibly circulating in great apes and other animals such as forest antelope in the Democratic Republic of the Congo (DRC), the Congo, Gabon, and perhaps elsewhere. Before the outbreak of Ebola in the Congo in the early 2000s, researchers documented a die-off in great apes. There was also an outbreak of the virus recorded in chimpanzees in Taï National Park in Ivory Coast. So before their recent expedition, Leendertz and his colleagues thought maybe humans had contracted Ebola in the latest outbreak from eating infected bushmeat, specifically, larger animals such as antelopes and apes. “People going into the forest have a higher likelihood to find more dead animals,” which they often take home and eat, Leendertz says.
But almost as soon as Leendertz arrived in Guinea, he realized the “large wildlife” hypothesis didn’t hold water: the ape populations in the forests of southern Guinea didn’t appear to be declining. In addition, the region around Meliandou is forest-free. “It’s just flat fields,” Leendertz says. “It is a long way to reach the first forest where you could expect larger wildlife . . . too far to have any likelihood of fresh bushmeat arriving in the village,” he explains. “The large wildlife hypothesis could quite clearly be excluded.” Then the scientists learned about the bat-filled tree that stood just 50 meters from Meliandou.
The idea that insectivorous bats could be a reservoir for Ebola isn’t far-fetched. Bats are particularly notorious for carrying zoonotic diseases, with more associated viruses than any other mammalian species. And researchers have successfully linked Ebola’s relative, Marburg virus, to a bat reservoir. In 2007, researchers isolated Marburg virus from Egyptian fruit bats (Rousettus aegyptiacus) living in a DRC gold mine near where 154 individuals, most of them miners, had been infected.2 Five years later, another set of scientists found that in Python Cave, a popular attraction in Uganda’s Queen Elizabeth National Park, roughly 2.5 percent of the well-studied R. aegyptiacus colony was actively infected with Marburg virus at any given time.3
In the early 2000s, Eric Leroy of the International Center for Medical Research in Gabon and his colleagues set out to identify Ebola’s reservoir. They collected and screened blood and tissue samples from more than 1,000 bats, birds, and rodents in Gabon and the Congo for traces of Ebola and found viral RNA fragments and antibodies against the virus in three bat species (fruit bats, not insectivores, in this case). However, the viral load—the quantity of the virus in the blood and tissue of each bat— was too low to isolate and replicate the virus, so the team couldn’t definitively say that the bats were the reservoir of Ebola.4 More than 10 years later, researchers still don’t know which species carry the virus, Leendertz says. “We are truly still fishing in the dark.”
Even when researchers successfully identify one possible reservoir of a zoonotic disease, that doesn’t mean there aren’t more. Since 1976, scientists have considered the Natal multimammate mouse (often called the African soft-furred rat) to be the sole reservoir of Lassa virus, which causes a sometimes fatal illness characterized by fever, headaches, vomiting, muscle pains, and potentially permanent hearing loss. “People get infected when the rodents come into houses and raid grain supplies,” explains Raina Plowright, an infectious disease ecologist and wildlife veterinarian at Montana State University. The mice leave virus-infected urine or feces in the house, and people contract the pathogen when they touch the excrement or inhale virus particles lingering in the air. But even as many individuals heed public health directives to store food in rodent-proof containers and take out the trash, the virus “spills over and kills thousands of people every year in West Africa,” Plowright says, suggesting there may be other reservoirs that transmit the virus, possibly in different ways.
Indeed, in 2016 Elisabeth Fichet-Calvet of the Bernhard Nocht Institute for Tropical Medicine in Germany and her colleagues published findings showing that the African wood mouse (Hylomyscus pamfi) in Nigeria and the Guinea multimammate mouse (Mastomys erythroleucus) in both Nigeria and Guinea also carry the Lassa virus.5 H. pamfi prefers the woods over fields, M. natalensis’s preferred habitat, and therefore the details of transmission to humans might differ, Fichet-Calvet and her colleagues note in their paper.
There have been hints that Ebola virus lurks in populations of large African wildlife, with an ongoing epidemic possibly circulating in great apes and other animals such as forest antelope in the Democratic Republic of the Congo (DRC), the Congo, Gabon, and perhaps elsewhere. Before the outbreak of Ebola in the Congo in the early 2000s, researchers documented a die-off in great apes. There was also an outbreak of the virus recorded in chimpanzees in Taï National Park in Ivory Coast. So before their recent expedition, Leendertz and his colleagues thought maybe humans had contracted Ebola in the latest outbreak from eating infected bushmeat, specifically, larger animals such as antelopes and apes. “People going into the forest have a higher likelihood to find more dead animals,” which they often take home and eat, Leendertz says.
But almost as soon as Leendertz arrived in Guinea, he realized the “large wildlife” hypothesis didn’t hold water: the ape populations in the forests of southern Guinea didn’t appear to be declining. In addition, the region around Meliandou is forest-free. “It’s just flat fields,” Leendertz says. “It is a long way to reach the first forest where you could expect larger wildlife . . . too far to have any likelihood of fresh bushmeat arriving in the village,” he explains. “The large wildlife hypothesis could quite clearly be excluded.” Then the scientists learned about the bat-filled tree that stood just 50 meters from Meliandou.
The idea that insectivorous bats could be a reservoir for Ebola isn’t far-fetched. Bats are particularly notorious for carrying zoonotic diseases, with more associated viruses than any other mammalian species. And researchers have successfully linked Ebola’s relative, Marburg virus, to a bat reservoir. In 2007, researchers isolated Marburg virus from Egyptian fruit bats (Rousettus aegyptiacus) living in a DRC gold mine near where 154 individuals, most of them miners, had been infected.2 Five years later, another set of scientists found that in Python Cave, a popular attraction in Uganda’s Queen Elizabeth National Park, roughly 2.5 percent of the well-studied R. aegyptiacus colony was actively infected with Marburg virus at any given time.3
In the early 2000s, Eric Leroy of the International Center for Medical Research in Gabon and his colleagues set out to identify Ebola’s reservoir. They collected and screened blood and tissue samples from more than 1,000 bats, birds, and rodents in Gabon and the Congo for traces of Ebola and found viral RNA fragments and antibodies against the virus in three bat species (fruit bats, not insectivores, in this case). However, the viral load—the quantity of the virus in the blood and tissue of each bat—was too low to isolate and replicate the virus, so the team couldn’t definitively say that the bats were the reservoir of Ebola.4 More than 10 years later, researchers still don’t know which species carry the virus, Leendertz says. “We are truly still fishing in the dark.”
Three out of four emerging infectious diseases are spread to humans from other animals.
Even when researchers successfully identify one possible reservoir of a zoonotic disease, that doesn’t mean there aren’t more. Since 1976, scientists have considered the Natal multimammate mouse (often called the African soft-furred rat) to be the sole reservoir of Lassa virus, which causes a sometimes fatal illness characterized by fever, headaches, vomiting, muscle pains, and potentially permanent hearing loss. “People get infected when the rodents come into houses and raid grain supplies,” explains Raina Plowright, an infectious disease ecologist and wildlife veterinarian at Montana State University. The mice leave virus-infected urine or feces in the house, and people contract the pathogen when they touch the excrement or inhale virus particles lingering in the air. But even as many individuals heed public health directives to store food in rodent-proof containers and take out the trash, the virus “spills over and kills thousands of people every year in West Africa,” Plowright says, suggesting there may be other reservoirs that transmit the virus, possibly in different ways.
“It’s just amazing that something that’s such a huge burden on health . . . we know so little about,” Plowright says.
Genetics, ecology, and other factors
In addition to some contact with a reservoir species—for example, via its meat, excrement, saliva, or blood—for zoonotic diseases to spill over into humans, the pathogen must be a generalist. “With exposure comes the chance an animal virus will jump to humans,” says Edward Holmes, an evolutionary biologist and virologist at the University of Sydney in Australia. “Then, it’s a question of whether that virus can infect human cells, and whether it can replicate to sufficient levels and have a route of transmission to the next host.” The same is true for nonviral zoonoses.
Being capable of infecting many different host species gives zoonotic pathogens a higher chance of survival. With a variety of hosts and environments to explore, bacteria, viruses, and other infectious agents can invade new niches. This concept helps explain why zoonotic pathogens leap from animal to animal before jumping to humans, as in the case of Hendra virus, which lurks in bats, is then transmitted to horses, and then to humans. But for that transmission to be successful, the virus has to replicate to levels that cause infection in each of the animals involved. And what allows that to happen isn’t well understood.
Researchers estimate that 631,000 to 827,000 unidentified viruses exist that have zoonotic potential.
Host immunity is likely one factor affecting susceptibility to infection and the likelihood of carrying a pathogen for long enough to transmit it to others; and researchers have documented immune differences among species. Humans infected with immunodeficiency virus (HIV), for example, tend to have greater proliferation of T cells than sooty mangabeys chronically infected with the related simian immunodeficiency virus (SIV). HIV-infected people also have a higher expression of genes activated by cell-signaling proteins called interferons than monkeys with SIV.6,7 The muted immune response in monkeys may prevent death of infected cells, allowing the virus to stay in the body and continue to replicate, and therefore have a chance to jump to a new host without the monkey succumbing to infection.
Researchers have also seen similar differences between monkeys that develop acquired immunodeficiency syndrome and those that don’t.8 “The innate immune response is different,” immunologist Judith Mandl of McGill University in Montreal tells The Scientist. Monkeys that develop AIDS produce more interferons and continue to produce them as the infection lingers, but monkeys that don’t develop AIDS somehow shut down the interferon response. “That first response to infection sets the scene for what happens after,” Mandl says—though scientists don’t yet understand what causes this difference in the first place.BAT FEVER: The flying mammals are notorious for harboring pathogens with zoonotic potential, such as Ebola virus, Hendra virus, and Marburg virus. In fact, bats have more associated viruses than any other mammalian species.© ISTOCK.COM/CRAIGRJD
Tools for studying the immune responses of animals other than humans, apes, and mice are limited, but researchers are now beginning to probe these factors in other potential reservoir species. For example, interferons may also play a role in bats’ incredible tolerance to viral infection: specifically, a mutation in the stimulator of interferon genes (STING), a signaling molecule that detects DNA floating in the cell cytoplasm. When STING senses free DNA in other mammals, the body generates interferons that spur an immune response to infection. But in bats, the mutation dampens interferon signaling and the consequent immune response to infection. And rodents appear to be able to tolerate hantavirus thanks to regulatory T cells that reduce effector T-cell response to the infection as it progresses, allowing the virus to persist. As a result, the virus continues to circulate in the body until an opportunity arises to move to another host, potentially humans.
See “Why Bats Make Such Good Viral Hosts”
Ecological factors offer other insights into the risk of zoonotic spillover. In the case of Hendra, for example, 20 years of data suggest that sudden shifts from long, dry spells to sudden wet ones can increase the prevalence of the virus in flying foxes (bats of the genus Pteropus). In a shift from dry to wet conditions, eucalyptus and gum trees, which produce flowers that bats feed on, suddenly put all of their energy into growth rather than reproduction. Fewer flowers means less nectar for bats. Facing starvation, the bats will search for food around farms and other places with livestock and humans, all while excreting the virus at high levels.9 Horses then contract the virus and spread it to humans. “Anecdotally, veterinarians have said there’s Hendra when it’s raining,” Plowright says.
Tracking bats’ behavior is lending weight to this idea. In recent years, for example, the northeastern Australian coast faced a long, dry El Niño that then changed to a wetter La Niña. In 2017, when Plowright and her colleagues were in Australia to track Hendra, the bats “were emaciated, just starving, and they were abandoning their young,” she says. “And we thought, ‘Well, we think we’re going to have a Hendra event,’ and we had a Hendra event.”
PATHS TO SPILLOVER: Zoonotic pathogens spread from animals to humans, and sometimes from humans back to animals. Mapping out the step-by-step pathway different zoonotic pathogens follow could help with surveillance efforts designed to prevent outbreaks.
See full infographic: WEB | PDF© LUCY CONKLIN
Sighting future outbreaks
A primary goal of zoonotic disease prediction is identifying where the next big outbreak will occur. But such prediction requires data, which the Cary Institute’s Han and her colleagues found to be lacking. “If we’re going be able to predict anything, anything in any market—weather or stock prices or real estate, anything like that—you need a background level of information,” Han says. For animal-borne diseases, “there was no baseline of where these things lived, and which species carried what, and so we realized we just needed to map everything,” she says.
Combining data on species diversity and zoonotic disease hosts, she and her colleagues identified the tropics, specifically South America and Eastern Africa, and some parts of Europe and the subarctic as hotspots for zoonotic disease outbreak.10 “One of the things that really jumped out to me was this pattern of where the carnivores are located,” Han says. “There’s lots of arctic carnivores [including foxes, wolves, and polar bears] and . . . we noticed that they carried more diseases than we would expect.” With climate change, the speed at which warming is happening in the Arctic is faster than elsewhere, and changes to the environment would bring changes to the disease dynamics there. “What’s going to happen when things thaw out, and humans start to make better use of that land that’s accessible since there’s no permafrost? That whole system is going to be really interesting to keep an eye on,” she says.
On a more local scale, understanding the factors that affect transmission could help scientists prevent outbreaks. In Kenya, for example, researchers have tied Rift Valley Fever with El Niño rainfall. The disease is caused by a virus that can infect livestock, which can then pass the pathogen on to humans. Mosquitoes, which rely on moisture to reproduce, prey on both livestock and humans and can also transmit the virus between species. In the past few years, researchers, veterinarians, and farmers have worked together to build a surveillance network to report symptoms of the virus in farm animals. Reports of miscarriages and hemorrhagic disease among livestock were highest in months with the highest rainfall, Harry Oyas of the Kenya Ministry of Agriculture and his colleagues wrote in April in PLOS Neglected Tropical Diseases.11 The early warning system developed in Kenya could prevent spillover of the Rift Valley Fever virus into humans, the authors say.
The program in Kenya is one of many monitoring programs developing around the world. In addition, researchers have launched several surveillance initiatives to detect and discover pathogens that have the potential to cause pandemics. A few of these projects, such as the University of California, Davis–based PREDICT program, have been capturing and testing zoonotic disease reservoir species to identify the pathogens they carry and determine their likelihood of jumping to humans. Some such efforts are focusing on known threats, such as MERS, SARS, and Nipah virus, but there are also pathogens that could have zoonotic potential that haven’t yet been identified.
Even with a growing understanding of how species leaps happen, precise outbreak predictions aren’t guaranteed.
Focusing on viruses, which have caused devastating epidemics in the last few decades, a team of researchers recently analyzed virus-host interactions, the history of viral zoonoses, and patterns of viral emergence, estimating that 631,000 to 827,000 unidentified viruses exist that have zoonotic potential.12 As a preemptive strike, the researchers have built on the PREDICT model and developed the estimated $1 billion Global Virome Project, which will collect blood samples from rodents, nonhuman primates, bats, and other wildlife to discover the majority of unknown viruses, with the aim of predicting the next pandemic—before it hits humans.
Not everyone is on board with the idea of predicting zoonotic disease outbreaks, however. The whole idea of pandemic prediction is “foolish,” Holmes tells The Scientist by email. “There are no generalities that can be used to make accurate predictions,” he says. Just because “you see a virus in animals does not mean it will spread in humans.” Rather than trying to predict the future, he supports “real-time proactive surveillance of the human-animal interface to see what we’re being exposed to.” Then, he says, with a globally coordinated response and research network, any emerging infection “can be stamped out quickly.”
References
- A.M. Saéz et al., “Investigating the zoonotic origin of the West African Ebola epidemic,” EMBO Mol Med, 7:17-23, 2015.
- R. Swanepoel et al., “Studies of reservoir hosts for Marburg virus,” Emerg Infect Dis, 13:1847-51, 2007.
- B.R. Amman et al., “Seasonal pulses of Marburg virus circulation in juvenile Rousettus aegyptiacus bats coincide with periods of increased risk of human infection,” PLOS Pathog, 8:e1002877, 2012.
- E.M. Leroy et al., “Fruit bats as reservoirs of Ebola virus,” Nature, 438:575-76, 2005.
- A. Olayemi et al., “New hosts of the Lassa virus,” Sci Rep, 6:25280, 2016.
- A. Kaur et al., “Diverse host responses and outcomes following simian immunodeficiency virus SIVmac239 infection in sooty mangabeys and rhesus macaques,” J Virol, 72:9597-611, 1998.
- S. Moir et al., “Pathogenic mechanisms of HIV disease,” Annu Rev Pathol, 6:223-48, 2011.
- B. Jacquelin et al., “Nonpathogenic SIV infection of African green monkeys induces a strong but rapidly controlled type I IFN response,” J Clin Invest, 119:3544-55, 2009.
- R.K. Plowright et al., “Reproduction and nutritional stress are risk factors for Hendra virus infection in little red flying foxes (Pteropus scapulatus),” Proc R Soc B, 275:861-69, 2008.
- B.A. Han et al., “Global patterns of zoonotic disease in mammals,” Trends Parasitol, 32:565-77, 2016.
- H. Oyas et al. “Enhanced surveillance for Rift Valley Fever in livestock during El Niño rains and threat of RVF outbreak, Kenya, 2015-2016,” PLOS Negl Trop Dis, 12:e0006353, 2018.
- D. Carroll et al., “The global virome project,” Science, 359:872-74, 2018.
Interested in reading more?
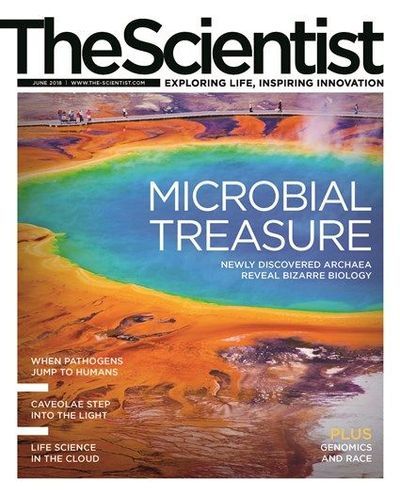