ABOVE: © ISTOCK.COM, DR_MICROBE
EDITOR’S CHOICE IN MICROBIOLOGY
One of the fundamental challenges all organisms face is how to survive in a dynamic environment. Bacteria, in particular, may find themselves awash with food one day and starving the next. Now, a team from biologist Lucy Shapiro’s lab at Stanford University has revealed a novel way that the bacterium Caulobacter crescentus, which reproduces in a stalked form attached to a substrate, regulates its cell growth and division, even when it’s in a state of famine: it forms a droplet-like membraneless organelle, or condensate, within its cytoplasm, collecting enzymes together in a way that enhances their ability to use ATP, the molecular fuel powering cell division.
During starvation, when ATP runs low, the bacterium forms a single condensate through a process called liquid-liquid phase separation (LLPS), similar to oil separating out atop a vinaigrette. The bacterium concentrates a cell division enzyme called DivJ inside the condensate. This allows for more efficient use of ATP to activate DivJ, which keeps the cell multiplying at regular intervals. When the researchers prevented a cell’s condensate from forming, either by removing the unstructured region of a protein called SpmX that’s involved in their formation or by using lipoic acid to inhibit phase separation, the local DivJ concentration was too low to effectively utilize ATP and promote cell division. In those cases, the bacterium grew longer but could not divide properly. When a bacterium has ample nutrition, abundant ATP prevents an unneeded condensate from forming.
Condensates have been documented in about a dozen bacterial systems to date, but experts tell The Scientist that the function of LLPS remains mostly enigmatic. The current study raises the question of whether condensate formation is ATP-dependent in other bacteria too, says Julie Biteen, a chemist and biophysicist at the University of Michigan who was not involved in the study. “I think [this study] makes it a very compelling hypothesis,” she says.
Biteen praises the variety of techniques the researchers used to pin down how C. crescentus regulates the activity of DivJ in response to environmental cues. “It’s such a nice synergy of techniques that really complement each other,” she says.
Shapiro and her colleagues used super-resolution microscopy to observe where condensates formed in live bacterial cells. At the same time, they used single particle tracking to observe the motion of DivJ molecules within the condensates. Then, the researchers froze the cells so they could look at condensates under a cryo-electron microscope, which captured nanometer-scale resolution images. This allowed them to observe the exact contours of a condensate and confirm which molecules were located inside and outside it. “That’s what I love about this,” Biteen says.
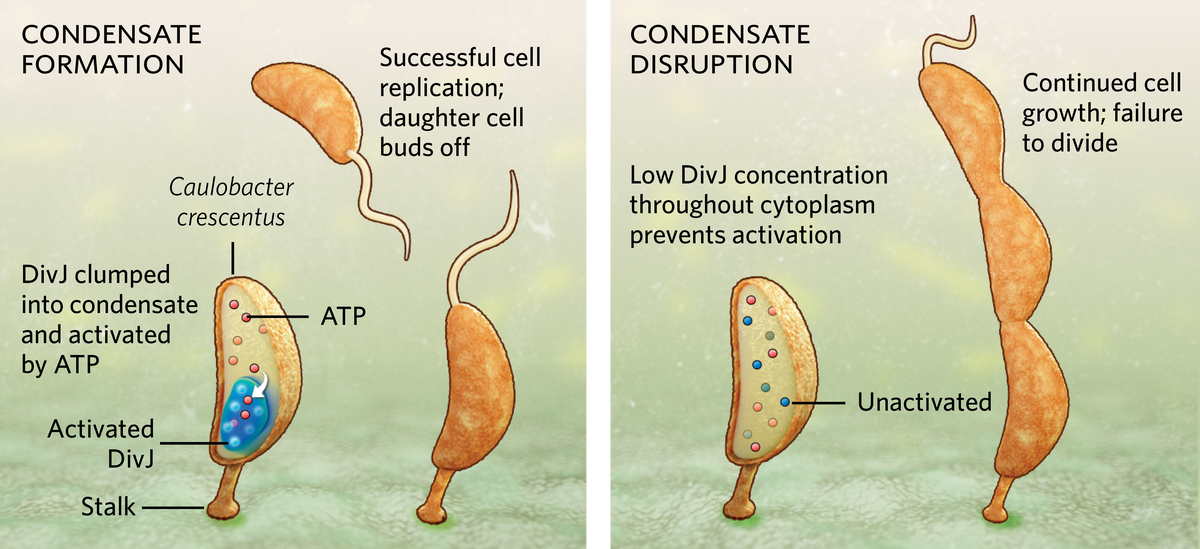
“Ours is the first study where we have shown a definitive function for phase separating condensates in bacterial adaptation,” explains lead study author Saumya Saurabh, a physical chemist who just launched his own lab at New York University but was affiliated with Stanford University at the time. He finds the mechanism to be elegant, he adds, because phase separation happens almost instantaneously when ATP levels drop below a certain point, and the process does not require energy input from the cell, unlike stress-triggered changes in gene expression that require energy and time to activate.
S. Saurabh et al., “ATP-responsive bio-molecular condensates tune bacterial kinase signaling,” Sci Adv, 8:eabm6570, 2022.