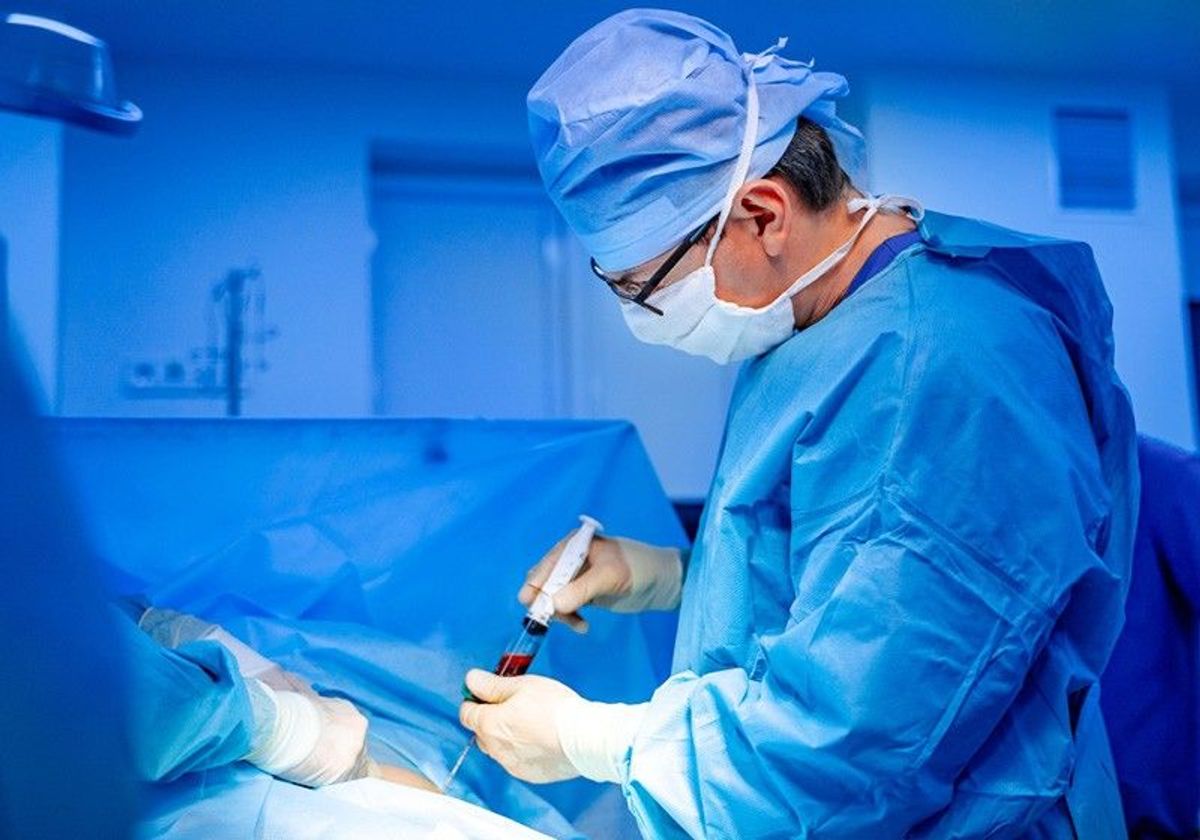
Stay up to date on the latest science with Brush Up Summaries.
What Are Hematopoietic Stem Cells?
Hematopoietic stem cells (HSCs) are multipotent progenitor cells found in the blood and bone marrow. They have the ability to self-renew, and during bone marrow hematopoiesis, HSCs differentiate into multiple cell types. Clinicians use HSCs to replace or repopulate a patient’s blood as a form of regenerative medicine or stem cell therapy. Research into HSC development and aging facilitates better in vitro HSC expansion and broadens their potential for disease treatment, enhancing their clinical therapeutic effects.
How Do HSCs Develop?
HSCs begin their development during embryogenesis in the dorsal aortic tissue and are additionally found in the placenta, yolk sac, and fetal liver. This fetal hematopoiesis process is necessary to produce the blood cells required for tissue development while generating a pool of undifferentiated HSCs. At birth, these HSCs migrate into and populate the newly-formed bone marrow and maintain a steady state of self-renewal and differentiation.1 Differentiation of hematopoietic stem cells produces red blood cells, platelets, and white blood cells throughout life, maintaining their levels following bleeding and infection. HSCs generally give rise to partially differentiated but proliferative progenitors, which differentiate into mature cells. Because of this process, true HSCs are relatively rare in the human body.2
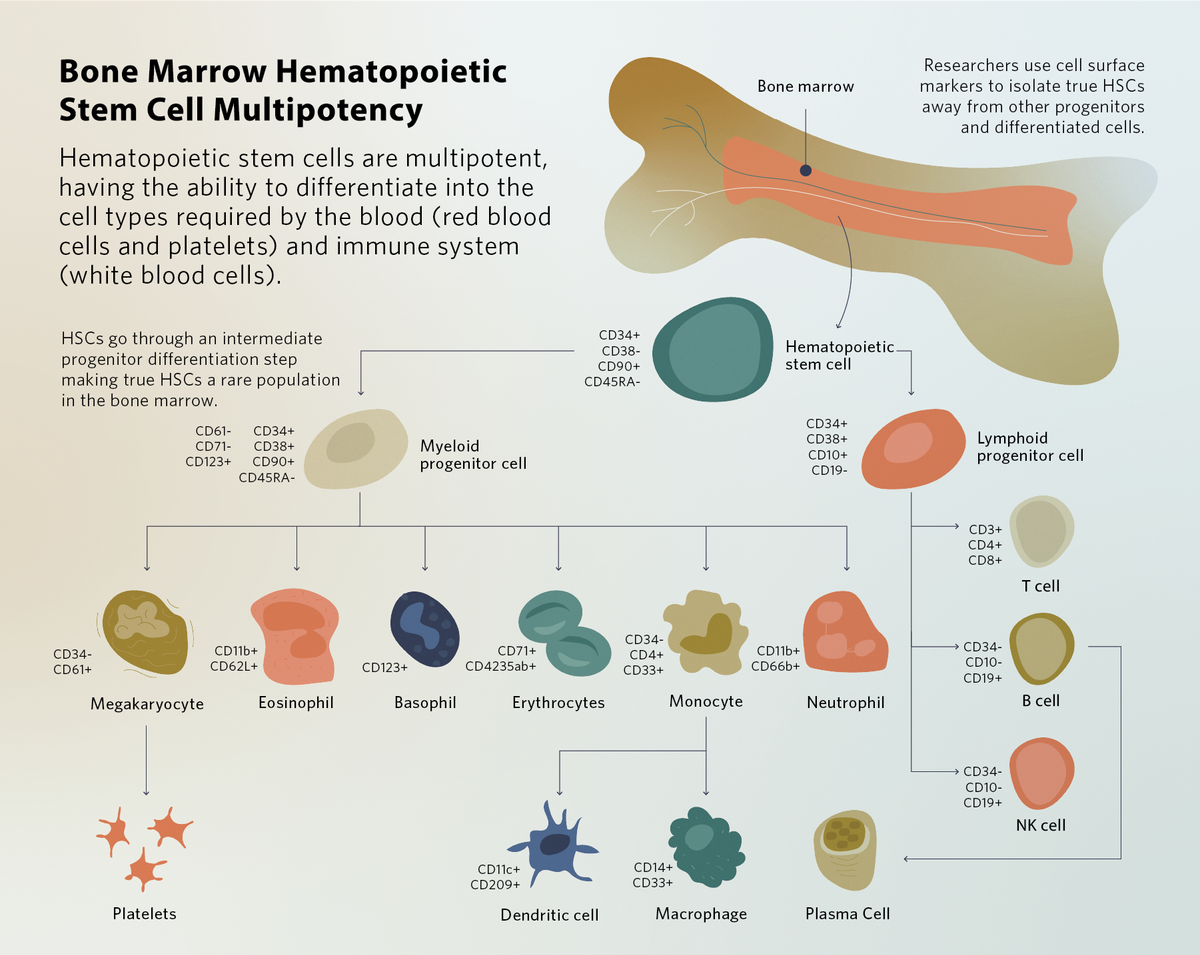
Using HSCs for Research and Treatment
Hematopoietic stem cell transplants
For more than 60 years, hematopoietic stem cell transplants (HSCTs) have been the most common form of HSC therapy, and are a standard option for treating hematologic malignancies, immunodeficiency, and defective hematopoiesis disorders. HSCs are now derived from multiple sources, such as peripheral blood, umbilical cord blood, and bone marrow. Before transplantation, the receiving patient must undergo severe immunosuppressive procedures to prevent rejection of the new stem cells.3
The most common HSC isolation method involves removing blood cells from plasma using density gradient centrifugation followed by magnetic bead isolation. Using flow cytometry, scientists sort specific HSC cell types based on common cell surface markers. The CD34 surface marker is a general marker for all hematopoietic progenitors, which enables researchers to physically capture CD34+ cells for stem cell therapy.4 Clinicians then intravenously infuse these cells into the receiver patient’s marrow, where the HSCs engraft and repopulate the blood and immune system. In blood cancers such as leukemias and lymphomas, restoration of the blood system by HSCT allows patients to receive high-dose chemotherapy treatments, ridding them of malignant cells. In patients with red blood cell conditions where continuous blood transfusions are not an option, such as thalassemia major, HSCT results in 80 percent disease-free survival.5
HSCs in regenerative medicine
Bone marrow HSCs also produce cells of other lineages, such as endothelial cells, cardiomyocytes, neural cells, and hepatocytes, in a process called transdifferentiation. Because adult stem cells are rare, understanding the mechanisms behind HSC transdifferentiation could provide an additional source of tissue-specific multipotent cells and influence future clinical methods for tissue regeneration. HSCs can also help repair injured organs by releasing regenerative cytokines and recruiting cells to the damage site.5
Delivering gene therapies with HSCs
Some of the latest advances in HSC therapeutic research involve using methods such as CRISPR for correcting genetically-defective HSCs, such as for sickle cell anemia and β-thalassaemia.6 These methods allow a patient to receive their own genetically-compatible (syngeneic) HSCs. These are called allogeneic transplants and are more effective at avoiding graft-versus-host disease, a condition where transplants from a donor are rejected by the recipient’s body, leading to an immune response against other tissues and organs. Creating genetically-corrected induced pluripotent stem cells (iPSCs) from patient skin tissues and differentiating them into HSCs has also been an active area of research, although current methods remain costly and time-consuming.7 Advances in HSC expansion protocols and genetic engineering may eventually allow researchers to mass-produce a single genetically engineered HSC population to serve as an off-the-shelf HSC therapy suitable for numerous patients. However, many challenges related to immunogenicity and safety remain.6 Further research is necessary to take advantage of these remarkable multipotent cells in disease therapies.
See also "The Future of Gene Therapy for a Rare Pediatric Autoimmune Disease"
Current and future therapeutic applications for HSCs
Disorders currently treated with HSCTs8 |
|
Future treatable diseases with HSCs |
|
Mobilizing HSCs for chemotherapy-free transplants
Compared to immature blood cells, HSCs have a higher potential to pass the bone marrow barrier, traveling in the blood from one bone to another. While traveling, HSCs can settle in other organs, such as the thymus, liver, or spleen, where they may develop into other specialized cells, such as T cells. This motility enables researchers and clinicians to harvest HSCs directly from the blood and opens opportunities for stem cell therapies that do not require genotoxic conditioning before transplant.11
See also "Death by Stem Cell: Developing New Cancer Therapies"
HSCs stay in the bone marrow or egress into peripheral blood based on the local niche microenvironment. To increase the number of circulating HSCs, scientists modulate niche components and properties, such as cytokines, growth factors, hormones, and extracellular matrix adhesion. Clinicians use HSC mobilization treatments, including granulocyte colony-stimulating factor, to increase the amount of circulating stem cells in donors. Because stem cell therapy engraftment involves resident and transplanted cells competing for space in the bone marrow niche, HSC mobilization in stem cell therapy recipients is also a growing area of interest. Rather than treating recipients with chemotherapy or total body radiation to evict resident cells from the niche before transplant, researchers aim to improve engraftment by sending resident cells on a niche vacation with HSC mobilization treatments. Experiments in mice have achieved promising results, but more work is needed to establish the safety and efficacy of HSC mobilization in humans.11
References
- Mikkola HK, Orkin SH. The journey of developing hematopoietic stem cells. Development. 2006;133(19):3733-44.
- Crane GM, et al. Adult haematopoietic stem cell niches. Nat Rev Immunol. 2017;17(9):573-90.
- Giralt S, Bishop MR. Principles and overview of allogeneic hematopoietic stem cell transplantation. Cancer Treat Res. 2009;144:1-21.
- Kumar B, Madabushi SS. Identification and isolation of mice and human hematopoietic stem cells. Methods Mol Biol. 2018;1842:55-68.
- Lee JY, Hong SH. Hematopoietic stem cells and their roles in tissue regeneration. Int J Stem Cells. 2020;13(1):1-12.
- Charlesworth CT, Hsu I, Wilkinson AC, Nakauchi H. Immunological barriers to haematopoietic stem cell gene therapy. Nat Rev Immunol. 2022;22(12):719-33.
- Demirci S, et al. Hematopoietic stem cells from pluripotent stem cells: Clinical potential, challenges, and future perspectives. Stem Cells Transl Med. 2020;9(12):1549-57.
- Khaddour K, et al. Hematopoietic stem cell transplantation. In: StatPearls [Internet]. 2023. Accessed September 18, 2023.
- Mahmud S, et al. Opportunities and challenges in stem cell therapy in cardiovascular diseases: Position standing in 2022. Saudi Pharm J. 2022;30(9):1360-71.
- Liu D, et al. Stem cells: a potential treatment option for kidney diseases. Stem Cell Res Ther. 2020;11(1):249.
- Canarutto D, et al. Mobilization-based engraftment of haematopoietic stem cells: a new perspective for chemotherapy-free gene therapy and transplantation. Br Med Bull. 2023;147(1):108-20.
This article was updated for clarity on September 21st, 2023
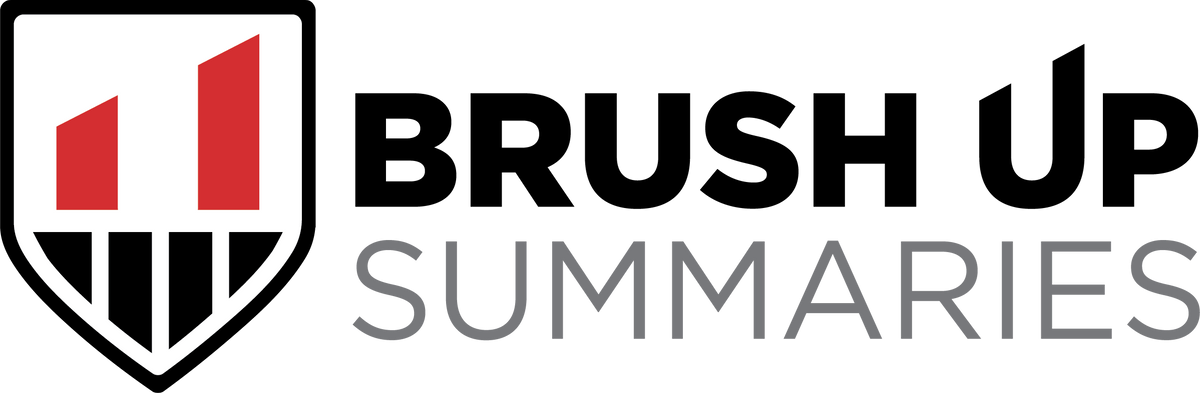