ABOVE: MODIFIED FROM © ISTOCK.COM, FILO
For the past few years, a biotech company called Tessera Therapeutics has been working away with a singular, lofty goal in mind: revolutionizing the technology used to edit DNA and RNA for scientific or therapeutic purposes.
The company is developing a suite of technologies it calls Gene Writing, all intended to expand the range of possible insertions, deletions, or edits that can be made to genetic material, while reducing the number of off-target alterations produced by more-traditional methods such as CRISPR-Cas9. The company claims that its techniques to “rewrite” or “write into” the genome without cleaving the DNA molecule, will help usher in a new era of highly effective, specific, and mechanistically simple gene editing. However, Tessera has shared precious little data with the world, and the approach that it’s championing is riddled with technical hurdles, raising questions among some researchers about whether the company can deliver what it’s promised.
Since CRISPR-Cas9 gene editing was first described in 2012, scientists have grown increasingly competent at editing, inserting, or deleting specific stretches of an organism’s DNA. In the early years, the most tried-and-true approach was to harness the abilities of natural or engineered nucleases—such as various Cas enzymes, transcription activator-like effector nucleases (TALENs), and zinc fingers—to break both strands of DNA’s signature double helix. Such breaks can be difficult for the cell to repair, however, leaving the genome vulnerable to errors in addition to the alterations researchers want to make. Newer and gentler approaches have since emerged in which the brute force of a nuclease’s double-strand breaks is replaced by single-strand breaks (also known as nicks) or sometimes no breaks at all, greatly increasing the efficiency and precision of gene edits.
Some successes of this new generation of genome-editing technologies include prime editing and base editing, which involve nicking DNA with a Cas nuclease and relying partly on the cell’s own DNA repair machinery to make precise changes. But researchers are limited in the types of changes they can make using these technologies—base editing, for example, can currently only substitute purine bases for other purines or pyrimidines for pyrimidines. Tessera instead plans to use mobile genetic elements (MGEs)—stretches of genetic material that are thought to make up half the human genome and are capable of moving around that genome without making double-strand breaks. In theory, explains company cofounder and board chair Geoffrey von Maltzahn, this could allow researchers to swap any individual base pair for any other.
For all of its investor interest, Tessera remains something of an enigma to researchers within both academia and industry.
It’s far from the only company to try to make this technology a reality. Massachusetts-based SalioGen Therapeutics, which closed a $115 million Series B financing round in January, is testing an MGE-based gene therapy platform for clinical applications that it calls Gene Coding. And Integra, based in Barcelona, Spain, has conducted preclinical tests of a similar MGE system, also dubbed Gene Writing, first described last December in Nature Communications. In March, Integra completed a roughly $6.3 million seed round of government and private funding. “We are continuously improving the technology,” says Marc Güell, cofounder and chief scientific officer of Integra Therapeutics. “Last year we had the year of base editing, prime editing. . . . I think this year will be the year of gene writing.”
See: “Adapting with a Little Help from Jumping Genes”
Yet Tessera seems to have stolen the spotlight. Spun out of the biotech venture capital company Flagship Pioneering in 2018, the company issued a press release about its trademarked Gene Writing technology—a trio of techniques that von Maltzahn says all use MGEs—in 2020. “At the outset of Tessera . . . we didn’t have clear perspectives as to how fruitful this endeavor was going to be,” von Maltzahn tells The Scientist, adding that the team shared a belief “that putting a lot of chips in the exact same place that Mother Nature put a bunch of chips was probably a worthwhile endeavor to do.”
Tessera placed its chips and a windfall of investor cash followed. Seven months after raising $2.7 million in a 2020 seed round, Tessera collected another $230 million in a January 2021 Series B, with Flagship increasing its financial stake alongside outside contributions. Earlier this year, Tessera completed its Series C, raising more than $300 million of additional funding, much of which came from the same cadre of investors. At the time, von Maltzahn told FierceBiotech that the company would continue “aggressively investing” in its platform and hoped to have operational technology by October 2023.
That funding has helped the company recruit skilled scientists who are well-regarded in the gene editing community. Dana Carroll, a University of Utah molecular biologist who helped pioneer early nuclease-based editors and previously licensed that tech to Sangamo Therapeutics, tells The Scientist that he recognizes a few of the scientists on Tessera’s team and that he respects their earlier work. “They got some experienced entrepreneurs in the biotech space, they’ve got some good scientists on board, and they got a lot of money,” Carroll says. “So, if they have some good ideas, the prospects may be bright.”
Tessera is simultaneously developing and testing three categories of Gene Writing systems—DNA Gene Writers, RNA Gene Writers, and RNA Gene Rewriters.
However, for all of its investor interest, Tessera remains something of an enigma to researchers within both academia and industry who spoke to The Scientist. The company has yet to publish peer-reviewed research or a white paper on its technology. Instead, it has primarily communicated progress through the occasional conference presentation, an approach that has caught the attention of prominent members of the community but left many questions unanswered—and some scientists skeptical.
“They’ve been on all of our radars through the grapevine,” University of California, San Diego, gene editing researcher Alexis Komor—a coauthor on the 2016 Nature paper first describing base editing and a former consultant at Beam Therapeutics, which commercialized that technology—says of academics’ perspective on Tessera. “We’re always like, ‘What are they doing, exactly?’ But no one actually knows. We’re all very interested.”
Three gene writers under development
Von Maltzahn tells The Scientist that Tessera is simultaneously developing and testing three categories of Gene Writing systems—DNA Gene Writers, RNA Gene Writers, and RNA Gene Rewriters—that are delivered to the target site inside a lipid nanoparticle. The company hopes the trio will ultimately form a comprehensive platform allowing for a wide variety of alterations ranging from single-base-pair substitutions to the insertion of entire genes.
For small alterations, there are the RNA Gene Rewriters, derived from MGEs called retrotransposons that essentially copy and paste their own sequences into new locations of a genome by means of an RNA intermediate and reverse transcription. Specifically, Tessera’s Rewriters use a process called target-primed reverse transcription, von Maltzahn explains, which involves writing the payload DNA sequence into a desired location by nicking one DNA strand at the target site, delivering an RNA template transcribed from the retrotransposon sequence, and assisting the cell’s transcription machinery in the reverse transcription of a complementary DNA strand. It’s these Rewriters that von Maltzahn says can outperform base editing by performing any kind of base pair substitution. They can also make substitutions, insertions, or deletions of up to roughly 100 base pairs in length, he says.
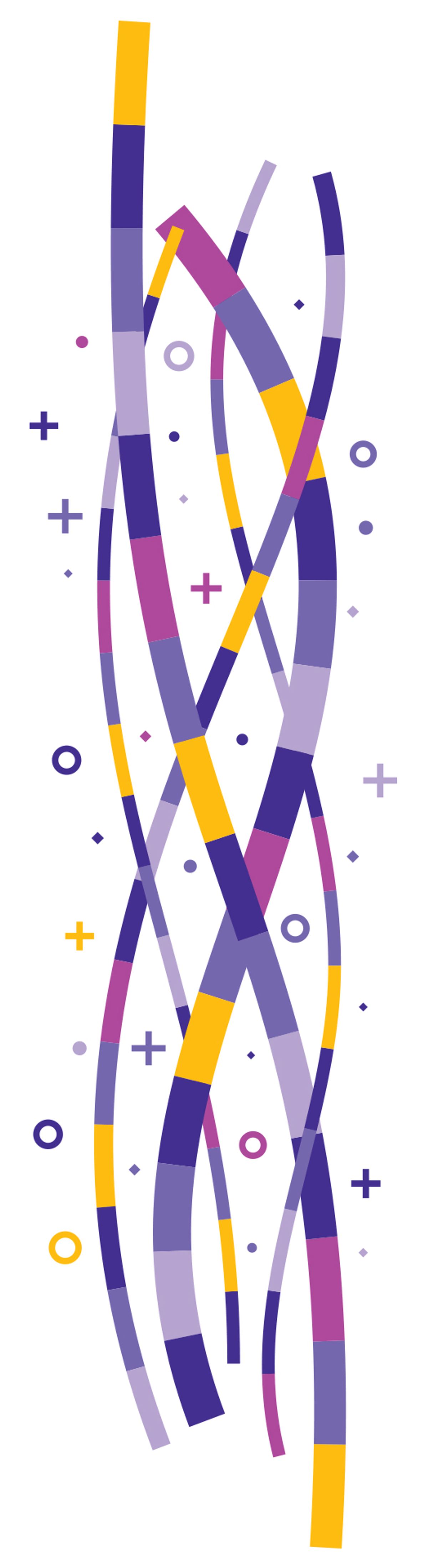
The other two systems are both designed to perform whole-gene insertions, vastly expanding the lengths of DNA that can be added by current cutting-edge techniques such as prime editing, which struggles to insert sequences longer than a few dozen base pairs. DNA Gene Writers are derived from recombinases, enzymes involved in the mobilization of MGEs that genetic engineers have used for decades and that are capable of rearranging or combining DNA sequences. With its own technology, Tessera aims to provide a DNA template that the recombinase would use to overwrite the DNA sequence at the target site via reverse transcription after inducing a single-strand break.
Then there are the RNA Gene Writers, which like the RNA Gene Rewriters are derived from retrotransposons, but which are capable of much longer insertions thanks to differences in the specific RNA template–encoding enzymes they contain. They include an RNA template and the RNA for that retrotransposon-encoding enzyme. “The RNA Gene Writer protein goes to the genome [and] nicks one strand, which leads to local unraveling, which leads to the hybridization of RNA bases to that location,” von Maltzahn says. “One letter at a time, it will write that RNA into DNA.”
See “In Editing RNA, Researchers See Endless Possibilities”
Von Maltzahn compares Tessera’s technology to existing CRISPR transposase systems that combine programmable MGEs with nucleases, as well as to piggyBac. The latter is a transposase-based gene delivery system that’s proven useful for in vitro and in vivo applications, but historically has run the risk of inserting DNA at unintended locations. Von Maltzahn also points to similarities between Tessera’s systems and naturally occurring transposons, some of which can insert their payload nearly anywhere in the genome. Like piggyBac, such transposons show a lack of specificity in insertion sites, and so have proven difficult to harness for gene editing therapies.
Part of the team’s work, von Maltzahn says, involves “brute force” testing of thousands of candidate MGEs to find sequences that precisely bind and deliver their payload to specific DNA sequences of interest—and then finding a way to reprogram them to deliver a desired or therapeutic payload sequence rather than whatever sequence they’d evolved to shuttle in nature. “Our endeavor at Tessera has been to continuously expand on top of the natural resource [that is] MGEs. We’ve tested over 20,000 MGEs inside of human cells at Tessera to date,” he says.
Initially, von Maltzahn tells The Scientist over email, Tessera was working with MGEs for RNA and DNA Gene Writers that more or less randomly insert their payload. However, the company has since managed to replace “the native DNA binding domain with a synthetic DNA binding domain,” which he says has allowed them to more precisely target desired integration sites. With the company’s RNA Gene Writers, “[a]s far as we know, we are the first to have shown that whole genes can be written into the genome merely by introducing two molecules of RNA into human cells,” he adds, clarifying that human cell testing is rare for the industry.
“I think large gene insertion can be interesting for a variety of diseases,” especially loss-of-function diseases such as spinal muscular atrophy, Duchenne muscular dystrophy, or cystic fibrosis, says Alexandra Urman, an analyst who covers therapeutics and the gene editing industry at ARK Investment Management. “And it’s a challenge that’s plagued gene editing in general.” If Tessera can figure out MGE gene editing that is specific, efficient, and programmable—and back up its claims with solid evidence—“that would be very interesting,” she adds.
Questions remain about Tessera’s technology
According to von Maltzahn, the company has kept its head down to work on developing and automating its technology—specifically running tests in human cells and animal models—but plans to publish in the future. Tessera shared very little data or findings on the performance of its trio of technologies with The Scientist, with von Maltzahn saying that the company has “prioritized filing intellectual property over scientific publications thus far.” The company did share a PowerPoint presentation that its scientists gave at the Precision Genome Engineering Keystone Symposia in April, describing how the company screened for MGEs that could target and overwrite mammalian genes and tested them in human cells.
Tessera also shared its presentation from the Federation of American Societies for Experimental Biology (FASEB) Genome Engineering Conference in June, in which the company provided some preliminary, preclinical information on its Gene Rewriters—a rare disclosure of specific data from a company that’s otherwise remained tight-lipped. The presentation suggested that Tessera could perform individual thymine-to-adenine conversions in target alleles with roughly 65 percent efficiency—20 times the rate at which it performed unintended or errant edits.
As is the case with many privately held companies, it is true that we have not yet shared much of our data publicly.
—Geoffrey von Maltzahn, Tessera Therapeutics
Still, the dearth of data and publications has raised more than a few eyebrows, as well as questions about whether Tessera’s technology will live up to the hype generated by the company’s financing. Urman, for example, expressed some hesitation about Tessera’s technology due to the lack of publications supporting its work. She tells The Scientist that she feels similarly to the way she did when writing a 2020 newsletter for ARK. Back then, she wrote: “Now Tessera Therapeutics . . . is claiming to write DNA without breaking it, something impossible to date without off target effects. Tessera uses mobile genetic elements (MGEs) to move or copy DNA into new locations, another technology that is not new.” She continued: “We believe other technologies will have to be supported by strong patents and data before they can compete [with any gene editing technology].”
Carroll and other researchers emphasize in particular the complexity of the challenge Tessera has taken on. Programming a therapeutic payload, combined with finding or programming MGEs to target a DNA sequence that’s specific enough to prevent MGEs from making the same edit throughout the human genome, has historically proven to be an all-but-insurmountable hurdle, experts tell The Scientist. Some MGEs “will just integrate [their payload] randomly throughout the genome everywhere,” says Komor. And even some of the more specific retrotransposons out there can still integrate into thousands of different genomic sites—far too imprecise for a therapeutically useful delivery system. Retrotransposons essentially “find a particular sequence and insert the RNA or DNA” on the spot, Komor explains. “And so, if that sequence happens to be the exact location of the genome where you want to do your editing, then that’s great. Chances are it’s not.”
Carroll notes that previous experiments with transposons by various researchers have found that “the enzymatic activity and the recognition activity are tightly linked within the proteins. So if you try to add some specificity by engineering, you’ll often decrease the efficiency. And if you try to increase the efficiency of it, you’ll decrease the specificity.” He also adds that those researchers typically avoided the headache by linking the transposon to a precision-boosting nuclease such as Cas9. “It has turned out to be extremely challenging and no one as far as I know has made it work,” Carroll says of the myriad attempts to use MGEs as gene editors, adding that he is hesitant to comment on Tessera’s research because of how little is known and because he hasn’t seen the company “give any references to the literature” that their work builds on.
Even when the audience at Tessera’s presentation at the Keystone Symposia pressed for more information on how—and how well—Tessera’s platform worked, they were left without many details about how the company was going to get around the issues associated with MGE editing, says Komor, who attended. “The talk at the conference was really exciting,” she says. “It was the first time we were going to see data.” However, she adds, very little information was presented, and “they had no actual information about the actual editor aside from that it uses RNA as a template.”
The presentation slides, shared with The Scientist by Tessera, do indicate that the company ran into problems with imprecise integration and had experimented with using Cas proteins to improve target site specificity. This use of Cas proteins is also a feature of existing gene editing techniques such as prime editing and, in an email to The Scientist, von Maltzahn refers to papers showing high site specificity in prime editing as a proof of concept for Tessera’s Gene Rewriters, since both use target-primed reverse transcription. In a follow-up email, von Maltzahn clarifies that Tessera’s technology differs from prime editing because, even though prime editing and Gene Rewriters both rely on a similar mechanism, “there are differences in the molecular machinery itself in both the template RNA and enzymatic protein” between the two technologies. Von Maltzahn additionally claims that Gene Rewriters can insert considerably longer stretches of DNA.
As for criticisms regarding a lack of information about Tessera’s technology, von Maltzahn responds: “As is the case with many privately held companies, it is true that we have not yet shared much of our data publicly, and this may limit the ability of some researchers who are not affiliated with Tessera to share informed opinions on our work.”
Nevertheless, even the more skeptical scientists who spoke to The Scientist say they remain hopeful that the challenges of MGE-based genome editing are on the way to being solved. After all, “nobody’s been able to get [CRISPR transposases] to work really well in mammalian cells,” Komor says, so it would be an exciting feat if Tessera has overcome the challenges associated with this or related approaches. Urman adds that if scientists believe in the promise of a technology, a proliferation of studies that use or validate it will follow in the next few years, as it has for CRISPR, base editing, and prime editing. Perhaps, she suggests, that phase for Gene Writing is still to come.